RESTORED: 3/11/22
Wow, the more research I do, the more I find that EVERYTHING is related. Follow along with me, it is a wild ride. God is revealing EVERYTHING that has been hidden. Line upon line, a little here, a little there.
There are several things that are getting clearer as I go. One is that BELGIUM is primary in this game. IF you have not seen my article on Belgium, check it out. BAAL – BEL-GIUM – LORD/OWNER/MASTER – ROYAL LINE – 10 Kingdoms
Another thing that is coming more and more clear is that ROCKS, more specifically QUARTZ also play a big role in this saga. I had no idea when I started my article about Rock CAIRNS where it would lead. Now, the rabbit hole is getting deeper.
And of course everyone can plainly see that computer/smartphones/the internet is the endgame. That is where we are all headed, to becoming wired to the mainframe and part of the machine. At least if they have their way. And that all started with SAND.
Hope you enjoy the article. I know it is long and I apologize for that….but there is so much to know.
38 Then the Lord answered Job out of the whirlwind, and said, 2 Who is this that darkeneth counsel by words without knowledge?
3 Gird up now thy loins like a man; for I will demand of thee, and answer thou me. 4 Where wast thou when I laid the foundations of the earth? declare, if thou hast understanding.
5 Who hath laid the measures thereof, if thou knowest? or who hath stretched the line upon it?
6 Whereupon are the foundations thereof fastened? or who laid the corner stone thereof; 7 When the morning stars sang together, and all the sons of God shouted for joy?
8 Or who shut up the sea with doors, when it brake forth, as if it had issued out of the womb? 9 When I made the cloud the garment thereof, and thick darkness a swaddlingband for it, 10 And brake up for it my decreed place, and set bars and doors, 11 And said, Hitherto shalt thou come, but no further: and here shall thy proud waves be stayed?
12 Hast thou commanded the morning since thy days; and caused the dayspring to know his place; 13 That it might take hold of the ends of the earth, that the wicked might be shaken out of it?
14 It is turned as clay to the seal; and they stand as a garment. 15 And from the wicked their light is withholden, and the high arm shall be broken.
16 Hast thou entered into the springs of the sea? or hast thou walked in the search of the depth?
17 Have the gates of death been opened unto thee? or hast thou seen the doors of the shadow of death?
18 Hast thou perceived the breadth of the earth? declare if thou knowest it all.
19 Where is the way where light dwelleth? and as for darkness, where is the place thereof, 20 That thou shouldest take it to the bound thereof, and that thou shouldest know the paths to the house thereof?
21 Knowest thou it, because thou wast then born? or because the number of thy days is great?
22 Hast thou entered into the treasures of the snow? or hast thou seen the treasures of the hail, 23 Which I have reserved against the time of trouble, against the day of battle and war?
24 By what way is the light parted, which scattereth the east wind upon the earth?
25 Who hath divided a watercourse for the overflowing of waters, or a way for the lightning of thunder; 26 To cause it to rain on the earth, where no man is; on the wilderness, wherein there is no man; 27 To satisfy the desolate and waste ground; and to cause the bud of the tender herb to spring forth?
28 Hath the rain a father? or who hath begotten the drops of dew?
29 Out of whose womb came the ice? and the hoary frost of heaven, who hath gendered it?
30 The waters are hid as with a stone, and the face of the deep is frozen.
31 Canst thou bind the sweet influences of Pleiades, or loose the bands of Orion?
32 Canst thou bring forth Mazzaroth in his season? or canst thou guide Arcturus with his sons?
33 Knowest thou the ordinances of heaven? canst thou set the dominion thereof in the earth?
34 Canst thou lift up thy voice to the clouds, that abundance of waters may cover thee?
35 Canst thou send lightnings, that they may go and say unto thee, Here we are?
36 Who hath put wisdom in the inward parts? or who hath given understanding to the heart?
37 Who can number the clouds in wisdom? or who can stay the bottles of heaven, 38 When the dust groweth into hardness, and the clods cleave fast together?
39 Wilt thou hunt the prey for the lion? or fill the appetite of the young lions, 40 When they couch in their dens, and abide in the covert to lie in wait?
41 Who provideth for the raven his food? when his young ones cry unto God, they wander for lack of meat. Job 38:4
If the foundations be destroyed, what can the righteous do? Psalm 11:3
The Wise and Foolish Builders
24 “Therefore everyone who hears these words of mine and puts them into practice is like a wise man who built his house on the rock. 25 The rain came down, the streams rose, and the winds blew and beat against that house; yet it did not fall, because it had its foundation on the rock. 26 But everyone who hears these words of mine and does not put them into practice is like a foolish man who built his house on sand. 27 The rain came down, the streams rose, and the winds blew and beat against that house, and it fell with a great crash.” Matthew 7:24-27
Sand – From Wikipedia, the free encyclopedia
![]() |
Sand dunes in the Idehan Ubari, Libya. |
![]() |
Depiction of glass sand, dune sand, quartz sand, volcanic sand, coral sand, garnet sand, and olivine sand. Samples are from Mongolia, Estonia, Hawaii, and mainland U.S. (1×1 cm each)[1] |
![]() |
Sand grains of yellow building sand. Microscope Lumam P-8. EPI lighting. The photo of each grain of sand is the result of multifocal stacking. |
Sand is a granular material composed of finely divided rock and mineral particles. It is defined by size, being finer than gravel and coarser than silt. Sand can also refer to a textural class of soil or soil type; i.e., a soil containing more than 85 percent sand-sized particles by mass.[2]
The composition of sand varies, depending on the local rock sources and conditions, but the most common constituent of sand in inland continental settings and non-tropicalcoastal settings is silica (silicon dioxide, or SiO2), usually in the form of quartz. The second most common type of sand is calcium carbonate, for example, aragonite, which has mostly been created, over the past half billion years, by various forms of life, like coral and shellfish. For example, it is the primary form of sand apparent in areas where reefs have dominated the ecosystem for millions of years like the Caribbean.
Sand is a non-renewable resource over human timescales, and sand suitable for making concrete is in high demand.[3] Desert sand, although plentiful, is not suitable for concrete. 50 billion tons of beach sand and fossil sand is used each year for construction.[4]
Sand grains of yellow building sand. Microscope Lumam P-8. EPI lighting. The photo of each grain of sand is the result of multifocal stacking.
- Abrasion: Before sandpaper, wet sand was used as an abrasive element between rotating devices with elastic surface and hard materials such as very hard stone (making of stone vases), or metal (removal of old stain before re-staining copper cooking pots).
- Agriculture: Sandy soils are ideal for crops such as watermelons, peaches, and peanuts, and their excellent drainage characteristics make them suitable for intensive dairy farming.
- Aquaria: Sand makes a low-cost aquarium base material which some believe is better than gravel for home use. It is also a necessity for saltwater reef tanks, which emulate environments composed largely of aragonite sand broken down from coral and shellfish.
- Artificial reefs: Geotextile bagged sand can serve as the foundation for new reefs.
- Artificial islands in the Persian Gulf.
- Beach nourishment: Governments move sand to beaches where tides, storms, or deliberate changes to the shoreline erode the original sand.[15]
- Brick: Manufacturing plants add sand to a mixture of clay and other materials for manufacturing bricks.[16]
- Cob: Coarse sand makes up as much as 75% of cob.
- Concrete: Sand is often a principal component of this critical construction material.
- Glass: Sand rich in silica is the principal component in common glasses.
- Hydraulic fracturing: A drilling technique for natural gas, which uses rounded silica sand as a “proppant”, a material to hold open cracks that are caused by the hydraulic fracturing process.
- Landscaping: Sand makes small hills and slopes (golf courses would be an example).
- Mortar: Sand is mixed with masonry cement or Portland cement and lime to be used in masonry construction.
- Paint: Mixing sand with paint produces a textured finish for walls and ceilings or non-slip floor surfaces.
- Railroads: Engine drivers and rail transit operators use sand to improve the traction of wheels on the rails.
- Recreation: Playing with sand is a favorite beach activity. One of the most beloved uses of sand is to make sometimes intricate, sometimes simple structures known as sand castles, proverbially impermanent. Special play areas for children, enclosing a significant area of sand and known as sandboxes, are common on many public playgrounds, and even at some single-family homes. Sand dunes are also popular among climbers, motorcyclists and beach buggy drivers.
- Roads: Sand improves traction (and thus traffic safety) in icy or snowy conditions.
- Sand animation: Performance artists draw images in sand. Makers of animated films use the same term to describe their use of sand on frontlit or backlit glass.
- Sand casting: Casters moisten or oil molding sand, also known as foundry sand and then shape it into molds into which they pour molten material. This type of sand must be able to withstand high temperatures and pressure, allow gases to escape, have a uniform, small grain size, and be non-reactive with metals.
- Sandbags: These protect against floods and gunfire. The inexpensive bags are easy to transport when empty, and unskilled volunteers can quickly fill them with local sand in emergencies.
- Sandblasting: Graded sand serves as an abrasive in cleaning, preparing, and polishing.
- Silicon: Quartz sand is a raw material for the production of silicon.
- Thermal weapon: While not in widespread use anymore, sand used to be heated and poured on invading troops in the classical and medieval time periods.
- Water filtration: Media filters use sand for filtering water
- Wuḍūʾ: an Islamic ritual wiping of parts of the body
- Zoanthid “skeletons”: Animals in this order of marine benthiccnidarians related to corals and sea anemones, incorporate sand into their mesoglea for structural strength, which they need because they lack a true skeleton.
Resources and environmental concerns
Only some sands are suitable for the construction industry, for example for making concrete. Because of the growth of population and of cities and the consequent construction activity there is a huge demand for these special kinds of sand, and natural sources are running low. In 2012 French director Denis Delestrac made a documentary called “Sand Wars“ about the impact of the lack of construction sand. It shows the ecological and economic effects of both legal and illegal trade in construction sand.[17][18][19]
To retrieve the sand, the method of hydraulic dredging is used. This works by pumping the top few meters of sand out of the water and filling it into a boat, which is then transported back to land for processing. Unfortunately, all marine life mixed in with the extracted sand is killed and the ecosystem can continue to suffer for years after the mining is complete. Not only does this affect marine life, but also the local fishing industries because of the loss of life, and communities living close to the water’s edge. When sand is taken out of the water it increases the risk of landslides, which can lead to loss of agricultural land and/or damage to dwellings.[20]
Sand’s many uses require a significant dredging industry, raising environmental concerns over fish depletion, landslides, and flooding.[21] Countries such as China, Indonesia, Malaysia, and Cambodia ban sand exports, citing these issues as a major factor.[22] It is estimated that the annual consumption of sand and gravel is 40 billion tons and sand is a US$70 billion global industry.[23]
The global demand for sand in 2017 was 9.55 billion tons as part of a $99.5 billion industry.[24]
Granite (/ˈɡrænɪt/) is a common type of felsicintrusiveigneous rock that is granular and phaneritic in texture. Granites can be predominantly white, pink, or gray in color, depending on their mineralogy. The word “granite” comes from the Latin granum, a grain, in reference to the coarse-grained structure of such a completely crystalline rock. Strictly speaking, granite is an igneous rock with between 20% and 60% quartz by volume, and at least 35% of the total feldspar consisting of alkali feldspar, although commonly the term “granite” is used to refer to a wider range of coarse-grained igneous rocks containing quartz and feldspar. Wikipedia
Quartz – From Wikipedia, the free encyclopedia
![]() MICA, QUARTZ.Galiléia, Minas Gerais, Brazil |
|
Category | oxide mineral[1][2] |
---|---|
Formula (repeating unit) |
SiO2 |
Strunz classification | 4.DA.05 (Oxides) |
Dana classification | 75.01.03.01 (tectosilicates) |
Crystal system | α-quartz: trigonal β-quartz: hexagonal |
Crystal class | α-quartz: trapezohedral (class 3 2); β-quartz: trapezohedral (class 6 2 2)[3] |
Unit cell | a = 4.9133 Å, c = 5.4053 Å; Z=3 |
Identification | |
Formula mass | 60.083 g·mol−1 |
Color | Colorless through various colors to black |
Crystal habit | 6-sided prism ending in 6-sided pyramid (typical), drusy, fine-grained to microcrystalline, massive |
Twinning | Common Dauphine law, Brazil law and Japan law |
Cleavage | {0110} Indistinct |
Fracture | Conchoidal |
Tenacity | Brittle |
Mohs scalehardness | 7 – lower in impure varieties (defining mineral) |
Luster | Vitreous – waxy to dull when massive |
Streak | White |
Diaphaneity | Transparent to nearly opaque |
Specific gravity | 2.65; variable 2.59–2.63 in impure varieties |
Optical properties | Uniaxial (+) |
Refractive index | nω = 1.543–1.545 nε = 1.552–1.554 |
Birefringence | +0.009 (B-G interval) |
Pleochroism | None |
Melting point | 1670 °C (β tridymite) 1713 °C (β cristobalite)[3] |
Solubility | Insoluble at STP; 1 ppmmass at 400 °C and 500 lb/in2 to 2600 ppmmass at 500 °C and 1500 lb/in2[3] |
Other characteristics | lattice: hexagonal, Piezoelectric, may be triboluminescent, chiral (hence optically active if not racemic) |
References | [1][4][5][6] |
Quartz is a hard, crystalline mineral composed of silicon and oxygen atoms. The atoms are linked in a continuous framework of SiO4 silicon–oxygen tetrahedra, with each oxygen being shared between two tetrahedra, giving an overall chemical formula of SiO2. Quartz is the second most abundant mineral in Earth‘s continental crust, behind feldspar.[7]
Quartz exists in two forms, the normal α-quartz and the high-temperature β-quartz, both of which are chiral. The transformation from α-quartz to β-quartz takes place abruptly at 573 °C (846 K; 1,063 °F). Since the transformation is accompanied by a significant change in volume, it can easily induce fracturing of ceramics or rocks passing through this temperature threshold.
There are many different varieties of quartz, several of which are semi-precious gemstones. Since antiquity, varieties of quartz have been the most commonly used minerals in the making of jewelry and hardstone carvings, especially in Eurasia.
SO, quartz is the mineral most often used to make Idols.
Etymology
The word “quartz” is derived from the German word “Quarz”, which had the same form in the first half of the 14th century in Middle High German in East Central German[8] and which came from the Polish dialect term kwardy, which corresponds to the Czech term tvrdý (“hard”).[9]
The Ancient Greeks referred to quartz as κρύσταλλος (krustallos) derived from the Ancient Greek κρύος (kruos) meaning “icy cold”, because some philosophers (including Theophrastus) apparently believed the mineral to be a form of supercooled ice.[10] Today, the term rock crystal is sometimes used as an alternative name for the purest form of quartz.
Crystal habit and structure
Quartz mineral embedded in limestone (top right of the sample), easily identifiable by its hexagonal form. It cannot be scratched by steel (see Mohs scale).
Quartz belongs to the trigonal crystal system. The ideal crystal shape is a six-sided prism terminating with six-sided pyramids at each end. In nature quartz crystals are often twinned (with twin right-handed and left-handed quartz crystals), distorted, or so intergrown with adjacent crystals of quartz or other minerals as to only show part of this shape, or to lack obvious crystal faces altogether and appear massive. Well-formed crystals typically form in a ‘bed’ that has unconstrained growth into a void; usually the crystals are attached at the other end to a matrix and only one termination pyramid is present. However, doubly terminated crystals do occur where they develop freely without attachment, for instance within gypsum. A quartz geode is such a situation where the void is approximately spherical in shape, lined with a bed of crystals pointing inward.
α-quartz crystallizes in the trigonal crystal system, space group P3121 or P3221 depending on the chirality. β-quartz belongs to the hexagonal system, space group P6222 and P6422, respectively.[11] These space groups are truly chiral (they each belong to the 11 enantiomorphous pairs). Both α-quartz and β-quartz are examples of chiral crystal structures composed of achiral building blocks (SiO4 tetrahedra in the present case). The transformation between α- and β-quartz only involves a comparatively minor rotation of the tetrahedra with respect to one another, without change in the way they are linked.
So we see that Quartz displays two very significant symbols of Satanist/Kabbalists/Illuminists/Magicians; the Tetrahedon and the CUBE.
Varieties (according to microstructure)
Although many of the varietal names historically arose from the color of the mineral, current scientific naming schemes refer primarily to the microstructure of the mineral. Color is a secondary identifier for the cryptocrystalline minerals, although it is a primary identifier for the macrocrystalline varieties.[12]
Major varieties of quartz Type Color & Description Transparent Herkimer diamond Colorless Transparent Rock crystal Colorless Transparent Amethyst Purple to violet colored quartz Transparent Citrine Yellow quartz ranging to reddish orange or brown, and occasionally greenish yellow Transparent Ametrine A mix of amethyst and citrine with hues of purple/violet and yellow or orange/brown Transparent Rose quartz Pink, may display diasterism Transparent Chalcedony Fibrous, variously translucent, cryptocrystalline quartz occurring in many varieties.
The term is often used for white, cloudy, or lightly colored material intergrown with moganite.
Otherwise more specific names are used.Carnelian Reddish orange chalcedony Translucent Aventurine Quartz with tiny aligned inclusions (usually mica) that shimmer with aventurescence Translucent to opaque Agate Multi-colored, curved or concentric banded chalcedony (cf. Onyx) Semi-translucent to translucent Onyx Multi-colored, straight banded chalcedony or chert (cf. Agate) Semi-translucent to opaque Jasper Opaque cryptocrystalline quartz, typically red to brown but often used for other colors Opaque Milky quartz White, may display diasterism Translucent to opaque Smoky quartz Light to dark gray, sometimes with a brownish hue Translucent to opaque Tiger’s eye Fibrous gold, red-brown or bluish colored chalcedony, exhibiting chatoyancy. Prasiolite Mint green Transparent Rutilated quartz Contains acicular (needle-like) inclusions of rutile Dumortierite quartz Contains large amounts of dumortierite crystals Blue Varieties (according to color)
Quartz crystal demonstrating transparency
Pure quartz, traditionally called rock crystal or clear quartz, is colorless and transparent or translucent, and has often been used for hardstone carvings, such as the Lothair Crystal. Common colored varieties include citrine, rose quartz, amethyst, smoky quartz, milky quartz, and others.[13] These color differentiations arise from the presence of impurities which change the molecular orbitals, causing some electronic transitions to take place in the visible spectrum causing colors. Polymorphs of quartz include: α-quartz (low), β-quartz, tridymite, moganite, cristobalite, coesite, and stishovite.
The most important distinction between types of quartz is that of macrocrystalline (individual crystals visible to the unaided eye) and the microcrystalline or cryptocrystalline varieties (aggregates of crystals visible only under high magnification). The cryptocrystalline varieties are either translucent or mostly opaque, while the transparent varieties tend to be macrocrystalline. Chalcedony is a cryptocrystalline form of silica consisting of fine intergrowths of both quartz, and its monoclinic polymorph moganite.[14] Other opaque gemstone varieties of quartz, or mixed rocks including quartz, often including contrasting bands or patterns of color, are agate, carnelian or sard, onyx, heliotrope, and jasper.
Amethyst
Amethyst is a form of quartz that ranges from a bright vivid violet to dark or dull lavender shade. The world’s largest deposits of amethysts can be found in Brazil, Mexico, Uruguay, Russia, France, Namibia and Morocco. Sometimes amethyst and citrine are found growing in the same crystal. It is then referred to as ametrine. An amethyst is formed when there is iron in the area where it was formed.
Blue quartz
Blue quartz contains inclusions of fibrous magnesio-riebeckite or crocidolite.[15]
Dumortierite quartz
Inclusions of the mineral dumortierite within quartz pieces often result in silky-appearing splotches with a blue hue, shades giving off purple and/or grey colors additionally being found. “Dumortierite quartz” (sometimes called “blue quartz”) will sometimes feature contrasting light and dark color zones across the material.[16][17] Interest in the certain quality forms of blue quartz as a collectible gemstone particularly arises in India and in the United States.[16]
Carnelian
Category Chalcedony variety Formula
(repeating unit)Silica (silicon dioxide, SiO2) Crystal system Trigonal Formula mass 60 g/mol Color Brownish-red Cleavage Absent Fracture Uneven, splintery, conchoidal Mohs scalehardness 6–7 Luster Vitreous, dull, greasy, silky Streak White Diaphaneity Translucent Specific gravity 2.59–2.61 References [1] Carnelian (also spelled cornelian[2]) is a brownish-red mineral commonly used as a semi-precious gemstone. Similar to carnelian is sard, which is generally harder and darker (the difference is not rigidly defined, and the two names are often used interchangeably). Both carnelian and sard are varieties of the silica mineral chalcedony colored by impurities of iron oxide. The color can vary greatly, ranging from pale orange to an intense almost-black coloration. It is most commonly found in Indonesia, Brazil, India, Russia (Siberia), and Germany.
Carnelian History
Carnelian intaglio with a Ptolemaic queen, Hellenistic artwork, Cabinet des Médailles Polish signet ring in light-orange carnelian intaglio showing Korwin coat of arms The red variety of chalcedony has been known to be used as beads since the Early Neolithic in Bulgaria. The first faceted (with constant 16+16=32 facets on each side of the bead) carnelian beads are described from the Varna Chalcolithic necropolis (middle of the 5th millennium BC).[3] The bow drill was used to drill holes into carnelian in Mehrgarh in the 4th-5th millennium BC.[4] Carnelian was recovered from Bronze AgeMinoan layers at Knossos on Crete in a form that demonstrated its use in decorative arts;[5] this use dates to approximately 1800 BC. Carnelian was used widely during Roman times to make engraved gems for signet or seal rings for imprinting a seal with wax on correspondence or other important documents. Hot wax does not stick to carnelian.[6] Sard was used for Assyriancylinder seals, Egyptian and Phoenicianscarabs, and early Greek and Etruscan gems.[7] The Hebrewodem (also translated sardius), the first stone in the High Priest’s breastplate, was a red stone, probably sard but perhaps red jasper.[7] In Revelation 4:3, the One seated on the heavenly throne seen in the vision of John the apostle is said to “look like jasper and ‘σαρδίῳ’ (sardius transliterated)”. And likewise it is in Revelation 21:20 as one of the precious stones in the foundations of the wall of the heavenly city.[8]There is a Neo-Assyrian seal made of carnelian in the Western Asiatic Seals collection of the British Museum that shows Ishtar–Gula as a star goddess. She is holding a ring of royal authority and is seated on a throne. She is shown with the spade of Marduk (his symbol), Sibbiti (שבע or sheva in Hebrew language) gods, stylus of Nabu and a worshiper. An 8th century BCE carnelian seal from the collection of the Ashmolean Museum shows Ishtar-Gula with her dog facing the spade of Marduk and his red dragon.[9]
Carnelian Etymology
Although now the more common term, “carnelian” is a 16th-century corruption of the 14th-century word “cornelian” (and its associated orthographies corneline and cornalyn).[10] Cornelian, cognate with similar words in several Romance languages, comes from the Mediaeval Latincorneolus, itself derived from the Latin word cornum, the cornel cherry,[11] whose translucent red fruits resemble the stone. The Oxford English Dictionary calls “carnelian” a perversion of “cornelian”, by subsequent analogy with the Latin word caro, carnis, flesh. According to Pliny the Elder, sard derived its name from the city of Sardis in Lydia from which it came, and according to others, may ultimately be related to the Persian word سرد sered, meaning yellowish red.[7]Sarx in Greek means “flesh”, and other stones have similar naming, such as the onyx stone in sardonx, which came from Greek for “claw” or “fingernail” because onyx with flesh-colored and white bands can resemble a fingernail.[12] So this type of use analogy may have been more widespread
Citrine
Citrine is a variety of quartz whose color ranges from a pale yellow to brown due to ferric impurities. Natural citrines are rare; most commercial citrines are heat-treated amethysts or smoky quartzes. However, a heat-treated amethyst will have small lines in the crystal, as opposed to a natural citrine’s cloudy or smokey appearance. It is nearly impossible to differentiate between cut citrine and yellow topaz visually, but they differ in hardness. Brazil is the leading producer of citrine, with much of its production coming from the state of Rio Grande do Sul. The name is derived from the Latin word citrina which means “yellow” and is also the origin of the word “citron“. Sometimes citrine and amethyst can be found together in the same crystal, which is then referred to as ametrine.[18] Citrine has been referred to as the “merchant’s stone” or “money stone”, due to a superstition that it would bring prosperity.[19]
Citrine was first appreciated as a golden-yellow gemstone in Greece between 300 and 150 BC, during the Hellenistic Age. The yellow quartz was used prior to that to decorate jewelry and tools but it was not highly sought after.[20]
Milky quartz
Milk quartz or milky quartz is the most common variety of crystalline quartz. The white color is caused by minute fluid inclusions of gas, liquid, or both, trapped during crystal formation,[21] making it of little value for optical and quality gemstone applications.[22]
Rose quartz
Rose quartz is a type of quartz which exhibits a pale pink to rose red hue. The color is usually considered as due to trace amounts of titanium, iron, or manganese, in the material. Some rose quartz contains microscopic rutile needles which produces an asterism in transmitted light. Recent X-ray diffraction studies suggest that the color is due to thin microscopic fibers of possibly dumortierite within the quartz.[23]
Additionally, there is a rare type of pink quartz (also frequently called crystalline rose quartz) with color that is thought to be caused by trace amounts of phosphate or aluminium. The color in crystals is apparently photosensitive and subject to fading. The first crystals were found in a pegmatite found near Rumford, Maine, US and in Minas Gerais, Brazil.[24]
Smoky quartz
Smoky quartz is a gray, translucent version of quartz. It ranges in clarity from almost complete transparency to a brownish-gray crystal that is almost opaque. Some can also be black. The translucency results from natural irradiation creating free silicon within the crystal.
Prasiolite
Prasiolite, also known as vermarine, is a variety of quartz that is green in color. Since 1950, almost all natural prasiolite has come from a small Brazilian mine, but it is also seen in Lower Silesia in Poland. Naturally occurring prasiolite is also found in the Thunder Bay area of Canada. It is a rare mineral in nature; most green quartz is heat-treated amethyst.[25]
Naturally occurring quartz crystals of extremely high purity, necessary for the crucibles and other equipment used for growing siliconwafers in the semiconductor industry, are expensive and rare. A major mining location for high purity quartz is the Spruce Pine Gem Mine in Spruce Pine, North Carolina, United States.[27] Quartz may also be found in Caldoveiro Peak, in Asturias, Spain.[28]
The largest documented single crystal of quartz was found near Itapore, Goiaz, Brazil; it measured approximately 6.1×1.5×1.5 m and weighed 39,916 kilograms.[29]
Safety
As quartz is a form of silica, it is a possible cause for concern in various workplaces. Cutting, grinding, chipping, sanding, drilling, and polishing natural and manufactured stone products can release hazardous levels of very small, crystalline silica dust particles into the air that workers breathe.[31] Crystalline silica of respirable size is a recognized human carcinogen and may lead to other diseases of the lungs such as silicosis and pulmonary fibrosis.[32][33]
History
The word “quartz” comes from the German
Quarz(help·info),[34] which is of Slavic origin (Czech miners called it křemen). Other sources attribute the word’s origin to the Saxon word Querkluftertz, meaning cross-vein ore.[35]
Quartz is the most common material identified as the mystical substance maban in Australian Aboriginal mythology. It is found regularly in passage tomb cemeteries in Europe in a burial context, such as Newgrange or Carrowmore in Ireland. The Irish word for quartz is grianchloch, which means ‘sunstone’. Quartz was also used in Prehistoric Ireland, as well as many other countries, for stone tools; both vein quartz and rock crystal were knapped as part of the lithic technology of the prehistoric peoples.[36]
While jade has been since earliest times the most prized semi-precious stone for carving in East Asia and Pre-Columbian America, in Europe and the Middle East the different varieties of quartz were the most commonly used for the various types of jewelry and hardstone carving, including engraved gems and cameo gems, rock crystal vases, and extravagant vessels. The tradition continued to produce objects that were very highly valued until the mid-19th century, when it largely fell from fashion except in jewelry. Cameo technique exploits the bands of color in onyx and other varieties.
Roman naturalist Pliny the Elder believed quartz to be water ice, permanently frozen after great lengths of time.[37] (The word “crystal” comes from the Greek word κρύσταλλος, “ice”.) He supported this idea by saying that quartz is found near glaciers in the Alps, but not on volcanic mountains, and that large quartz crystals were fashioned into spheres to cool the hands. This idea persisted until at least the 17th century. He also knew of the ability of quartz to split light into a spectrum.
In the 17th century, Nicolas Steno‘s study of quartz paved the way for modern crystallography. He discovered that regardless of a quartz crystal’s size or shape, its long prism faces always joined at a perfect 60° angle.[38]
Quartz’s piezoelectric properties were discovered by Jacques and Pierre Curie in 1880.[39][40] The quartz oscillator or resonator was first developed by Walter Guyton Cady in 1921.[41][42]George Washington Pierce designed and patented quartz crystal oscillators in 1923.[43][44][45] Warren Marrison created the first quartz oscillator clock based on the work of Cady and Pierce in 1927.[46]
Efforts to synthesize quartz began in the mid nineteenth century as scientists attempted to create minerals under laboratory conditions that mimicked the conditions in which the minerals formed in nature: German geologist Karl Emil von Schafhäutl (1803–1890)[47] was the first person to synthesize quartz when in 1845 he created microscopic quartz crystals in a pressure cooker.[48] However, the quality and size of the crystals that were produced by these early efforts were poor.[49]
By the 1930s, the electronics industry had become dependent on quartz crystals. The only source of suitable crystals was Brazil; however, World War II disrupted the supplies from Brazil, so nations attempted to synthesize quartz on a commercial scale. German mineralogist Richard Nacken (1884–1971) achieved some success during the 1930s and 1940s.[50] After the war, many laboratories attempted to grow large quartz crystals. In the United States, the U.S. Army Signal Corps contracted with Bell Laboratories and with the Brush Development Company of Cleveland, Ohio to synthesize crystals following Nacken’s lead.[51][52] (Prior to World War II, Brush Development produced piezoelectric crystals for record players.) By 1948, Brush Development had grown crystals that were 1.5 inches (3.8 cm) in diameter, the largest to date.[53][54] By the 1950s, hydrothermal synthesis techniques were producing synthetic quartz crystals on an industrial scale, and today virtually all the quartz crystal used in the modern electronics industry is synthetic.
Piezoelectricity
Some types of quartz crystals have piezoelectric properties; they develop an electric potential upon the application of mechanical stress.[56] An early use of this property of quartz crystals was in phonograph pickups. One of the most common piezoelectric uses of quartz today is as a crystal oscillator. The quartz clock is a familiar device using the mineral. The resonant frequency of a quartz crystal oscillator is changed by mechanically loading it, and this principle is used for very accurate measurements of very small mass changes in the quartz crystal microbalance and in thin-film thickness monitors.
spacer
Feldspar From Wikipedia, the free encyclopedia
![]() Feldspar crystal (18×21×8.5 cm)
from Jequitinhonha valley,
Minas Gerais, southeastern Brazil
|
|
Category | Tectosilicate |
---|---|
Formula (repeating unit) |
KAlSi3O8 – NaAlSi3O8 – CaAl2Si2O8 |
Crystal system | Triclinic or monoclinic |
Color | pink, white, gray, brown, blue |
Cleavage | two or three |
Fracture | along cleavage planes |
Mohs scale hardness | 6.0–6.5 |
Luster | Vitreous |
Streak | white |
Diaphaneity | opaque |
Specific gravity | 2.55–2.76 |
Density | 2.56 |
Refractive index | 1.518–1.526 |
Birefringence | first order |
Pleochroism | none |
Other characteristics | exsolution lamellae common |
References | [1] |
Feldspars (KAlSi3O8 – NaAlSi3O8 – CaAl2Si2O8) are a group of rock-forming tectosilicate minerals that make up about 41% of the Earth‘s continental crust by weight.[2][3]Feldspars crystallize from magma as both intrusive and extrusive igneous rocks and are also present in many types of metamorphic rock.[4] Rock formed almost entirely of calcic plagioclase feldspar is known as anorthosite.[5] Feldspars are also found in many types of sedimentary rocks.[6]
Etymology
The name feldspar derives from the German Feldspat, a compound of the words Feld (“field”) and Spat (“flake”). Spat had long been used as the word for “a rock easily cleaved into flakes”; Feldspat was introduced in the 18th century as a more specific term, referring perhaps to its common occurrence in rocks found in fields (Urban Brückmann, 1783) or to its occurrence as “fields” within granite and other minerals (René-Just Haüy, 1804).[16] The change from Spat to -spar was influenced by the English word spar,[17] meaning a non-opaque mineral with good cleavage.[18] Feldspathic refers to materials that contain feldspar. The alternate spelling, felspar, has fallen out of use. The term ‘felsic’, meaning light colored minerals such as quartz and feldspars, is an acronymic word derived from feldspar and silica, unrelated to the redundant spelling ‘felspar’.
Sand : The Never-Ending Story
From individual grains to desert dunes, from the bottom of the sea to the landscapes of Mars, and from billions of years in the past to the future, this is the extraordinary story of one of nature’s humblest, most powerful, and most ubiquitous materials. Told by a geologist with a novelist’s sense of language and narrative, Sand examines the science–sand forensics, the physics of granular materials, sedimentology, paleontology and archaeology, planetary exploration–and at the same time explores the rich human context of sand. Interwoven with tales of artists, mathematicians, explorers, and even a vampire, the story of sand is an epic of environmental construction and destruction, an adventure in staggering scales of time and distance, yet a tale that encompasses the ordinary and everyday. Sand, in fact, is all around us–it has made possible our computers, buildings and windows, toothpaste, cosmetics, and paper, and it has played dramatic roles in human history, commerce, and imagination. In this luminous, kinetic, revelatory account, we do indeed find the world in a grain of sand.
Let us start this message by paying tribute to Sibelco’s Honorary President Stanislas Emsens Sr., who passed away in January 2018 at the age of ninety-one. Acknowledged as the father of Sibelco, it is impossible to overstate Stany’s influence during 52 years of active management, in which time he led the incredible growth of Sibelco from a Belgian silica sand business to a truly international multi-mineral group. His forward-vision and dedication were an inspiration to all of us, and his wisdom and guidance will be sorely missed.
This has been a year of transformational change and significant progress for Sibelco as we made good headway in the implementation of the new strategy we defined towards the end of 2016. Working together as one global team, we achieved key milestones against each element of our vision to be the best global material solutions company; market-driven, operationally excellent, with a compelling culture.
The results of our efforts were reflected in an improved financial performance. Against 2016, EBITDA increased by 26% to €541m, whilst Net Cash Flow (before dividend and growth capex) was up nearly 49% to €371m. Revenue was €3.1bn compared with €2.7bn last year.
Belgian mining company Sibelco further expands in the US
Yesterday the American shale sand supplier Fairmount Santrol Holdings Inc announced a planned merge with Unimin Corp in a cash-and-stock deal. Unimin Corp is a wholly owned subsidiary of Belgian mining company SCR-Sibelco NV. The deal is expected to close by the middle of next year.
Unimin received financing from Barclays Bank and BNP Paribas for the merge. Together, the two companies will become one of the largest suppliers of sand for use in hydraulic fracturing to shale oil and gas producers with about $2 billion in annual revenue. Fairmount shareholders will receive $170 million in cash and ownership of about 35 percent of the new company, while Unimin will own the rest.
“Together we will serve our customers more efficiently and effectively with a broader and more diverse product offering, greater technical expertise, improved scale and geographic diversity and an expanded logistics platform,” Fairmount Chief Executive Jenniffer Deckard commented on the merge. Deckard is expected to be the CEO of the new company.
spacer
HOUSTON (Reuters) – Fairmount Santrol Holdings Inc said on Tuesday it would sell itself to Unimin Corp in a cash-and-stock deal to create one of the largest suppliers of sand for use in hydraulic fracturing to shale oil and gas producers.
The deal, which is expected to close by the middle of next year, will create a company with about $2 billion in annual revenue and will supply more than 45 million tons of sand annually. The new company will also supply industrial coatings.
Sand, known as proppant in the oil industry, is used as part of the fracking process to hold open tiny cracks in shale rock in order to allow hydrocarbon molecules to escape. Oil companies typically shop for various shapes and sizes of sand to suit their geology. That has made the sand industry appealing to investors in recent years.
“Together we will serve our customers more efficiently and effectively with a broader and more diverse product offering, greater technical expertise, improved scale and geographic diversity and an expanded logistics platform,” Fairmount Chief Executive Jenniffer Deckard said in a statement.
Deckard is expected to be CEO of the new company, which has not yet been named.
The combined company “should bring more discipline and pricing power,” Jefferies LLC oilfield service analyst Brad Handler wrote in a report on Tuesday. The merged business would be able to generate strong cash flow and pay down debt, which he estimated to be about $1.6 billion.
Fairmount shareholders will receive $170 million in cash and own about 35 percent of the new company after the deal closes. Unimin, a division of Belgium-based SCR-Sibelco NV, will own the rest.
Unimin received financing from Barclays Bank and BNP Paribas for the deal.
Reporting by Ernest Scheyder; Editing by Susan Thomas and Peter Cooney
spacer


Sep 12, 2018
Subterrene – From Wikipedia, the free encyclopedia
Trebelev subterrene (Soviet Union)
A subterrene (Latin: subterrina, Russian: Подземная лодка) is a vehicle that travels underground (through solid rock/soil) much as a submarine travels underwater, either by mechanical drilling, or by melting its way forward. Subterrenes existed first in fiction as mechanical drillers,[citation needed] with real-world thermal designs and examples following in the second half of the 20th century.
Fictional subterrenes are often depicted as cylindrical in shape with conical drill heads at one or both ends, sometimes with some kind of tank-tread for propulsion, and described either as leaving an empty tunnel behind it, or as filling the space behind it with mining debris such as the Thunderbirds Mole. The plausibility of such machines has declined with the advent of the real-world tunnel boring machines, which demonstrate the reality of the boring task. Tunnel boring machine themselves are not usually considered to be subterrenes, possibly because they lack the secondary attributes – mobility and independence – that are normally applied to vehicles.
A real-world, mobile subterrene must work thermally, using very high temperature and immense pressure to melt and push through rock. The front of the machine is equipped with a stationary drill tip which is kept at 1,300–1,700 °F (700–930 °C). The molten rock is pushed around the edges as the vehicle is forced forward, and cools to a glass-like lining of the tunnel. Massive amounts of energy are required to heat the drill head, supplied via nuclear power or electricity. Patents issued in the 1970s[1] indicate that U.S. scientists had planned to use nuclear power to liquefy lithium metal and circulate it to the front of the machine (drill). An onboard nuclear reactor can permit a truly independent subterrene, but cooling the reactor is a difficult problem.[2] The Soviet Union is purported to have built such a “battle mole”, which operated until its onboard reactor failed.[3]
On line information presents research that was funded by the United States Government for the Los Alamos Scientific Laboratories University of California, Los Alamos, New Mexico for a project Camelot under the heading Systems and Cost Analysis for a Nuclear Subterrene Tunneling Machine. A patent was subsequently issued under number 3,693,731 on September 26, 1972[4].
The design concepts fall into similar designs of current technology of our nuclear submarine fleet and existing tunnel boring technology as used in the Channel Tunnel between England and France, but with the added feature of melting rock for the tunnel wall lining.
THIS IS CLEAR PROOF THAT PHIL SCHNEIDER WAS TELLING THE TRUTH!!! EVERYTHING IN THE ABOVE ARCTICLE VERIFIES EVERY WORD HE SAID ABOUT THE TUNNELS, LOS ALAMOS, THE DRILLING MACHINE THAT LEAVES THE SMOOTH AS GLASS WALLS AS IT BORES THROUGH THE EARTH!!
This article possibly contains original research. (September 2009)
7 Giant Machines That Changed the World—And 1 That Might
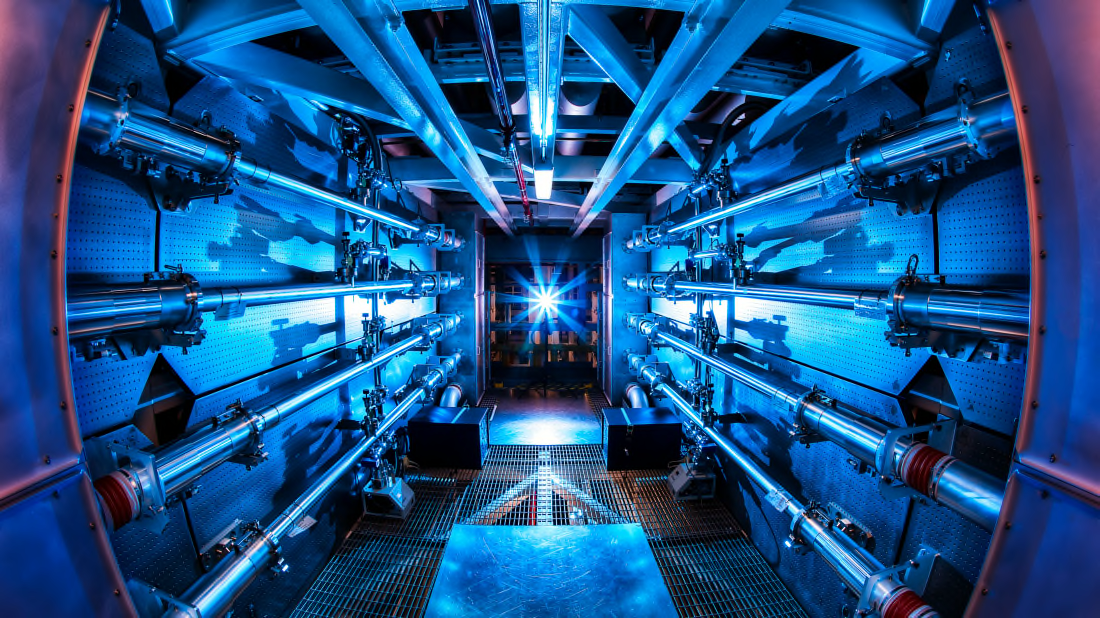
1. LARGE HADRON COLLIDER
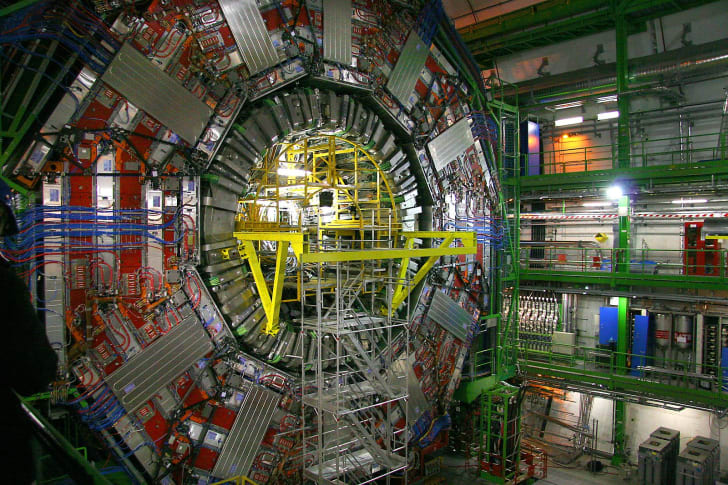
The Large Hadron Collider, a particle accelerator located at CERN outside of Geneva, Switzerland, is the largest machine in the world: It has a circumference of almost 17 miles and took around a decade to build. The tubes of the LHC are a vacuum; superconducting magnets guide and accelerate two high-energy particle beams, which are moving in opposite directions, to near-light-speed. When the beams collide, scientists use the data to find the answers to some of the most basic questions of physics and the laws that govern the universe we live in.
Since the LHC started up in 2008, scientists have made numerous groundbreaking discoveries, including finding the once-theoretical Higgs boson particle—a.k.a. the “God” particle—which helps give other particles mass. Scientists had been chasing the Higgs boson for five decades. The discovery illuminates the early development of the universe, including how particles gained mass after the Big Bang. Scientists are already working on the LHC’s successor, which will be three times its size and seven times more powerful.
2. CRAWLER-TRANSPORTER ROCKET MOVERS
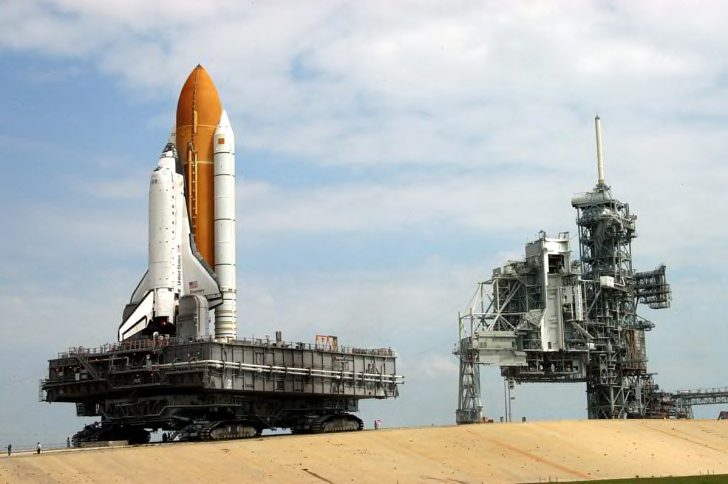
Built in 1965, NASA’s crawler-transporters are two of the largest vehicles ever constructed: They weigh 2400 tons each and burn 150 gallons of diesel per mile. In contrast, the average semi truck gets roughly 6.5 miles per gallon. The vehicles’ first job was to move Saturn V rockets—which took us to the moon and measured 35 stories tall when fully constructed—from the massive Vehicle Assembly Building (the largest single-room building in the world) to the launch pad at Cape Canaveral. The 4.2-mile trip was a slow one; the transporters traveled at a rate of 1 mph to ensure the massive rockets didn’t topple over. Without a vehicle to move rockets from the spot they were stacked to the launch pad, we never could have gotten off the ground, much less to the moon.
After our moon missions, the crawler-transporters were adapted to service the Space Shuttle program, and moved the shuttles from 1981 to 2003. Since the retirement of the orbiters, these long-serving machines are once again being repurposed to transport NASA’s new Space Launch System (SLS), which, at 38 stories tall, will be the biggest rocket ever constructed when it’s ready, hopefully in a few years (the timeline is in flux due to budgetary issues).
3. NATIONAL IGNITION FACILITY
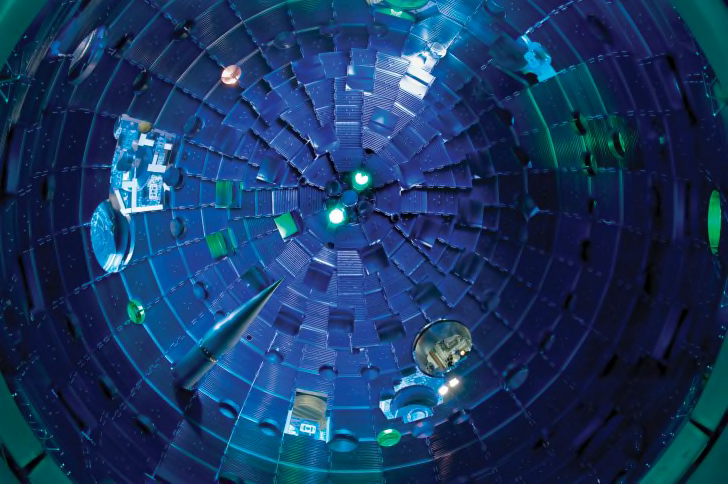
Three football fields could fit inside the National Ignition Facility, which holds the largest, most energetic, and most precise laser in the world (it also has the distinction of being the world’s largest optical instrument). NIF—which took about a decade to build and opened in 2009—is located at the Lawrence Livermore National Laboratory in Livermore, California. Its lasers are used to create conditions not unlike those within the cores of stars and giant planets, which helps scientists to gain understanding about these areas of the universe. The NIF is also being used to pursue the goal of nuclear fusion. If we can crack the code for this reaction that powers stars, we’ll achieve unlimited clean energy for our planet.
“Laser” is an acronym for light amplification by stimulated emission of radiation. A laser is created when the electrons in atoms in special glasses, crystals, or gases absorb energy from an electrical current or another laser and become “excited.” The excited electrons move from a lower-energy orbit to a higher-energy orbit around the atom’s nucleus. When they return to their normal or “ground” state, the electrons emit photons (particles of light).
These photons are all at the same wavelength and are “coherent,” meaning the crests and troughs of the light waves are all in lockstep. In contrast, ordinary visible light comprises multiple wavelengths and is not coherent.
Laser light is different from normal light in other ways as well. First, its light contains only one wavelength (one specific color). The particular wavelength of light is determined by the amount of energy released when the excited electron drops to a lower orbit. Second, laser light is directional. Whereas a laser generates a very tight beam, a flashlight produces light that is diffuse. Because laser light is coherent, it stays focused for vast distances, even to the moon and back.
spacer
Aug 13, 2019
Stay Tuned
In NIF, as in most large lasers, intense flashes of white light from giant flashlamps “pump” electrons in large slabs of laser glass to a higher-energy state that lasts only about one-millionth of a second. A small pulse of laser light “tuned” to the excited electrons’ energy is directed through the glass slabs. This laser pulse stimulates the electrons to drop to their lower, or ground, energy states and emit a laser photon of exactly the same wavelength.
An optical switch traps the low-energy laser pulse in the main amplifier for four passes through the laser glass slabs. Mirrors at both ends of the glass amplifier cause the photons to travel back and forth through the glass, stimulating more electrons to drop to their lower energy states and emit photons. This process produces huge numbers of photons of the same wavelength and direction—an extremely bright and straight beam of light. In this way the initial low-energy pulse is amplified by more than a quadrillion times to create 192 highly energetic, tightly focused laser beams that converge in the center of the Target Chamber.
Lasers Are Everywhere
Lasers can be tiny constituents of microchips or as immense as NIF, which is ten stories high and as wide as three football fields. Lasers are found in a dazzling range of products and technologies, including CD and DVD players, metal-cutting machines, measuring systems, and eye and cosmetic surgery. Early lasers could produce peak powers of some 10,000 watts. Modern lasers can produce pulses that are billions of times more powerful. Scientists have demonstrated NIF’s ability to generate more than 500 trillion watts of power.
Some lasers, such as ruby lasers, emit short pulses of light. Others, like helium–neon gas lasers or liquid dye lasers, emit light that is continuous. NIF, like the ruby laser, emits pulses of light lasting only billionths of a second.
Laser light does not need to be visible. NIF beams start out as invisible infrared light and then pass through special optics that convert them to visible green light and then to invisible, high-energy ultraviolet light for optimum interaction with the target.
4. BERTHA THE TUNNEL BORER
spacer
When Seattle decided it needed a giant tunnel to replace an aging highway through the middle of the city, the city contracted with Hitachi Zosen Corporation to build the biggest tunnel boring machine in the world to do the job. The scope of Bertha’s work had no precedent in modern-day digging, given the dense, abrasive glacial soil and bedrock it had to chew through.In 2013, Bertha—named after Bertha Knight Landes, Seattle’s first female mayor—was tasked with building a tunnel that would be big enough to carry four lanes of traffic (a two-lane, double-decker road). Bertha needed to carve through 1.7 miles of rock, and just 1000 feet in, the 57-foot, 6559-ton machine ran into a steel pipe casing that damaged it. Many predicted that Bertha was doomed, but after a massive, on-the-spot repair operation by Hitachi Zosen that took a year-and-a-half, the borer was up and running again.
In April 2017, Bertha completed its work, and engineers started the process of dismantling it; its parts will be used in future tunnel boring machines. Bertha set an example for what is possible in future urban tunnel work—but it’s unlikely that tunnel boring machines will get much bigger than Bertha because of the sheer weight of the machine and the amount of soil it can move at once. Bertha’s tunnel is scheduled to open in 2019.
DARPA Looks for Tunneling Breakthroughs

DARPA is funding breakthroughs in tunneling. The Underminer project aims to demonstrate the feasibility of rapidly constructing tactical tunnel networks to provide secure logistics infrastructure to pre-position supplies or resupply troops as they move through an area.
General Electric Research Center and Colorado School of Mines will focus on development of an integrated solution for Underminer technology and operational needs.
Colorado School of Mines has a web page dedicated to their new tunneling project.
TUNNEL BORING MACHINE AI
Mike Mooney and his Colorado School of Mines team are using AI to characterize ground conditions, learn how to tunnel really fast, and minimize tunneling-induced building deformation.
Tunnel boring machines (TBMs) excavate through highly uncertain and poorly characterized heterogeneous soil and rock. TBM interaction with the ground is very complex, with dozens of integrated processes governing the efficiency and effectiveness of tunnel construction. Such complex processes in highly unknown environments are ideal for AI intelligent learning, a process whereby the TBM learns-while-doing, continuously improving its performance to best achieve the tunneling objectives. In this research we are using artificial intelligence techniques and constrained optimization to learn and implement the best tunneling strategy for the encountered ground.
RAPID TUNNELING
There will be the development of rapid tunneling.
Boring Company Tunnel Machines
Elon Musks Boring Company is not directly involved in the DARPA project. They are leaders in developing faster tunneling.
The first three boring machines used by The Boring Company are:
Godot, a conventional tunnel boring machine made by Canadian company Lovat, which is used for research purposes.
Line-storm, a highly modified conventional boring machine. In February 2019, Elon Musk estimated in a tweet that Line-storm would be active “in a month or so”.
Prufrock is a BoringCompany designed machine. It is slated to support a 15x improvement in tunneling speed over the existing state of the art in 2017. It has the same diameter as a Dragon spacecraft (3.7 metres (12 ft)). In February 2020, Prufrock was shown assembled in a Twitter post.
Colorado School of Mines is also looking at Large-Diameter Tunnel Lining Design, Dynamic Risk Mapping and Midtown Immersed Tube Tunnel Analysis.
Sandia National Laboratories will conduct technology exploration and integration to address current process and system limitations.
The performers will focus on tunneling approaches, downhole sensing, and operations concepts. Underminer seeks to merge breakthroughs in horizontal drilling, trenchless boring technologies, and robotics to create a set of systems allowing consistent underground access.
Fast creation of tactical tunnels could benefit contingency operations such as rapid ammunition resupply, rescue missions and other functions.
Tunneling Industry
Recent years have seen significant advances in tunneling equipment and materials. TBM (Tunnel Boring Machines) and microtunneling equipment with enhanced data acquisitions, cutter/tool wear monitoring and replacement capabilities. All forms of tunnel lining — precast segmental, cast-in-place, and shotcrete — have benefited from advances in steel and synthetic fiber.
SOURCES- DARPA, Colorado School of Mines, Tunneling Online, Boring Company
Written By Brian Wang, Nextbigfuture.com
Tunnel Boring Machine a shitload of information on this site
spacer
5. INTERNATIONAL SPACE STATION
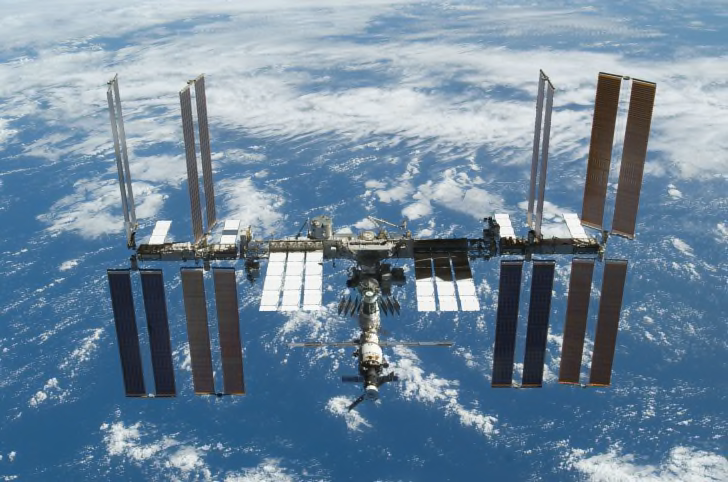
The international space station is a highly efficient machine, equipped with instrumentation and life support equipment, that has kept humans alive in the inhospitable environment of low-Earth orbit since November 2, 2000. It’s the biggest satellite orbiting the Earth made by humans. The major components were sent into space over a two-year period, but construction has slowly continued over the last decade, with astronauts adding the Columbus science laboratory and Japanese science module. The first module, Zarya, was just 41.2 feet by 13.5 feet; now, the ISS is 356 feet by 240 feet, which is slightly larger than a football field. The station currently has about 32,333 cubic feet of pressurized volume the crew can move about in. That’s about the same area as a Boeing 747 (though much of the ISS’s space is taken up by equipment). The U.S.’s solar panels are as large as eight basketball courts.
From the space station, scientists have made such important discoveries as what extended zero-G does to the human body, where cosmic rays come from, and how protein crystals can be used to treat cancer. Though NASA expects the most modern modules of the ISS to be usable well into the 2030s, by 2025 the agency may begin “transitioning” much of its ISS operations—and costs—to the private sector [PDF] with an eye on expanding the commercial potential of space.
6. LIGO GRAVITATIONAL WAVE DETECTOR
That looks exactly like the spiral in the sky over Norway…
What Is This Mysterious Spiral Anomaly in the Norwegian Sky?December 9, 2009 Norway awaiting the arrival of Barack Obama see a strange phenomenon arriving out of the eastern sky. You know the “spiral” is another symbol that is very significant to the Satanists/Kabbalists/Illuminati/Magicians.
The Laser Inferometer Gravitational-Wave Observatory (LIGO) is actually made up of four different facilities—two laboratories and two detectors located 2000 miles apart, in Hanford, Washington, and Livingston, Louisiana. The detectors, which took about five years to build and were inaugurated in 1999, are identical L-shaped vacuum chambers that are about 2.5 miles long and operate in unison. The mission of these machines is to detect ripples in the fabric of spacetime known as gravitational waves. Predicted in 1915 by Einstein’s theory of general relativity, gravitational waves were entirely theoretical until September 2015, when LIGO detected them for the first time. Not only did this provide further confirmation of general relativity, it opened up entirely new areas of research such as gravitational wave astronomy. The reason the two detectors are so far from each other is to reduce the possibility of false positives; both facilities must detect a potential gravitational wave before it is investigated.
Image credit: The SXS (Simulating eXtreme Spacetimes) Project
spacer
Gravitational Waves Detected 100 Years After Einstein’s Prediction
News Release • February 11, 2016
Visit The Detection Portal
See also: LIGO Hanford Press Release
LIGO Opens New Window on the Universe with Observation of Gravitational Waves from Colliding Black Holes
WASHINGTON, DC/Cascina, Italy
For the first time, scientists have observed ripples in the fabric of spacetime called gravitational waves, arriving at the earth from a cataclysmic event in the distant universe. This confirms a major prediction of Albert Einstein’s 1915 general theory of relativity and opens an unprecedented new window onto the cosmos.
Gravitational waves carry information about their dramatic origins and about the nature of gravity that cannot otherwise be obtained. Physicists have concluded that the detected gravitational waves were produced during the final fraction of a second of the merger of two black holes to produce a single, more massive spinning black hole. This collision of two black holes had been predicted but never observed.
SORRY, I CALL BULLS__T
The gravitational waves were detected on September 14, 2015 at 5:51 a.m. Eastern Daylight Time (09:51 UTC) by both of the twin Laser Interferometer Gravitational-wave Observatory (LIGO) detectors, located in Livingston, Louisiana, and Hanford, Washington, USA. The LIGO Observatories are funded by the National Science Foundation (NSF), and were conceived, built, and are operated by Caltech and MIT. The discovery, accepted for publication in the journal Physical Review Letters, was made by the LIGO Scientific Collaboration (which includes the GEO Collaboration and the Australian Consortium for Interferometric Gravitational Astronomy) and the Virgo Collaboration using data from the two LIGO detectors.
Based on the observed signals, LIGO scientists estimate that the black holes for this event were about 29 and 36 times the mass of the sun, and the event took place 1.3 billion years ago. About 3 times the mass of the sun was converted into gravitational waves in a fraction of a second—with a peak power output about 50 times that of the whole visible universe. By looking at the time of arrival of the signals—the detector in Livingston recorded the event 7 milliseconds before the detector in Hanford—scientists can say that the source was located in the Southern Hemisphere.
According to general relativity, a pair of black holes orbiting around each other lose energy through the emission of gravitational waves, causing them to gradually approach each other over billions of years, and then much more quickly in the final minutes. During the final fraction of a second, the two black holes collide into each other at nearly one-half the speed of light and form a single more massive black hole, converting a portion of the combined black holes’ mass to energy, according to Einstein’s formula E=mc2. This energy is emitted as a final strong burst of gravitational waves. It is these gravitational waves that LIGO has observed.
The existence of gravitational waves was first demonstrated in the 1970s and 80s by Joseph Taylor, Jr., and colleagues. Taylor and Russell Hulse discovered in 1974 a binary system composed of a pulsar in orbit around a neutron star. Taylor and Joel M. Weisberg in 1982 found that the orbit of the pulsar was slowly shrinking over time because of the release of energy in the form of gravitational waves. For discovering the pulsar and showing that it would make possible this particular gravitational wave measurement, Hulse and Taylor were awarded the Nobel Prize in Physics in 1993.
The new LIGO discovery is the first observation of gravitational waves themselves, made by measuring the tiny disturbances the waves make to space and time as they pass through the earth.
“Our observation of gravitational waves accomplishes an ambitious goal set out over 5 decades ago to directly detect this elusive phenomenon and better understand the universe, and, fittingly, fulfills Einstein’s legacy on the 100th anniversary of his general theory of relativity,” says Caltech’s David H. Reitze, executive director of the LIGO Laboratory.
The discovery was made possible by the enhanced capabilities of Advanced LIGO, a major upgrade that increases the sensitivity of the instruments compared to the first generation LIGO detectors, enabling a large increase in the volume of the universe probed—and the discovery of gravitational waves during its first observation run. The US National Science Foundation leads in financial support for Advanced LIGO. Funding organizations in Germany (Max Planck Society), the U.K. (Science and Technology Facilities Council, STFC) and Australia (Australian Research Council) also have made significant commitments to the project. Several of the key technologies that made Advanced LIGO so much more sensitive have been developed and tested by the German UK GEO collaboration. Significant computer resources have been contributed by the AEI Hannover Atlas Cluster, the LIGO Laboratory, Syracuse University, and the University of Wisconsin- Milwaukee. Several universities designed, built, and tested key components for Advanced LIGO: The Australian National University, the University of Adelaide, the University of Florida, Stanford University, Columbia University of the City of New York, and Louisiana State University.
“In 1992, when LIGO’s initial funding was approved, it represented the biggest investment the NSF had ever made,” says France Córdova, NSF director. “It was a big risk. But the National Science Foundation is the agency that takes these kinds of risks. We support fundamental science and engineering at a point in the road to discovery where that path is anything but clear. We fund trailblazers. It’s why the U.S. continues to be a global leader in advancing knowledge.”
LIGO research is carried out by the LIGO Scientific Collaboration (LSC), a group of more than 1000 scientists from universities around the United States and in 14 other countries. More than 90 universities and research institutes in the LSC develop detector technology and analyze data; approximately 250 students are strong contributing members of the collaboration. The LSC detector network includes the LIGO interferometers and the GEO600 detector. The GEO team includes scientists at the Max Planck Institute for Gravitational Physics (Albert Einstein Institute, AEI), Leibniz Universität Hannover, along with partners at the University of Glasgow, Cardiff University, the University of Birmingham, other universities in the United Kingdom, and the University of the Balearic Islands in Spain.
“This detection is the beginning of a new era: The field of gravitational wave astronomy is now a reality,” says Gabriela González, LSC spokesperson and professor of physics and astronomy at Louisiana State University.
LIGO was originally proposed as a means of detecting these gravitational waves in the 1980s by Rainer Weiss, professor of physics, emeritus, from MIT; Kip Thorne, Caltech’s Richard P. Feynman Professor of Theoretical Physics, emeritus; and Ronald Drever, professor of physics, emeritus, also from Caltech.
“The description of this observation is beautifully described in the Einstein theory of general relativity formulated 100 years ago and comprises the first test of the theory in strong gravitation. It would have been wonderful to watch Einstein’s face had we been able to tell him,” says Weiss.
“With this discovery, we humans are embarking on a marvelous new quest: the quest to explore the warped side of the universe—objects and phenomena that are made from warped spacetime. Colliding black holes and gravitational waves are our first beautiful examples,” says Thorne.
Virgo research is carried out by the Virgo Collaboration, consisting of more than 250 physicists and engineers belonging to 19 different European research groups: 6 from Centre National de la Recherche Scientifique (CNRS) in France; 8 from the Istituto Nazionale di Fisica Nucleare (INFN) in Italy; 2 in The Netherlands with Nikhef; the Wigner RCP in Hungary; the POLGRAW group in Poland; and the European Gravitational Observatory (EGO), the laboratory hosting the Virgo detector near Pisa in Italy. WOW, WHERE DOES ALL THIS MONEY COME FROM TO PAY ALL THESE GUYS AND MAINTAIN ALL THIS EQUIPMENT?
Fulvio Ricci, Virgo Spokesperson, notes that, “This is a significant milestone for physics, but more importantly merely the start of many new and exciting astrophysical discoveries to come with LIGO and Virgo.”
Bruce Allen, managing director of the Max Planck Institute for Gravitational Physics (Albert Einstein Institute), adds, “Einstein thought gravitational waves were too weak to detect, and didn’t believe in black holes. But I don’t think he’d have minded being wrong!”
“The Advanced LIGO detectors are a tour de force of science and technology, made possible by a truly exceptional international team of technicians, engineers, and scientists,” says David Shoemaker of MIT, the project leader for Advanced LIGO. “We are very proud that we finished this NSF-funded project on time and on budget.”
At each observatory, the two-and-a-half-mile (4-km) long L-shaped LIGO interferometer uses laser light split into two beams that travel back and forth down the arms (four-foot diameter tubes kept under a near-perfect vacuum). The beams are used to monitor the distance between mirrors precisely positioned at the ends of the arms. According to Einstein’s theory, the distance between the mirrors will change by an infinitesimal amount when a gravitational wave passes by the detector. A change in the lengths of the arms smaller than one-ten-thousandth the diameter of a proton (10-19 meter) can be detected.
“To make this fantastic milestone possible took a global collaboration of scientists—laser and suspension technology developed for our GEO600 detector was used to help make Advanced LIGO the most sophisticated gravitational wave detector ever created,” says Sheila Rowan, professor of physics and astronomy at the University of Glasgow.
Independent and widely separated observatories are necessary to determine the direction of the event causing the gravitational waves, and also to verify that the signals come from space and are not from some other local phenomenon.
Toward this end, the LIGO Laboratory is working closely with scientists in India at the Inter-University Centre for Astronomy and Astrophysics, the Raja Ramanna Centre for Advanced Technology, and the Institute for Plasma to establish a third Advanced LIGO detector on the Indian subcontinent. Awaiting approval by the government of India, it could be operational early in the next decade. The additional detector will greatly improve the ability of the global detector network to localize gravitational-wave sources.
“Hopefully this first observation will accelerate the construction of a global network of detectors to enable accurate source location in the era of multi-messenger astronomy,” says David McClelland, professor of physics and director of the Centre for Gravitational Physics at the Australian National University. ARE THEY KIDDING???
Additional video and image assets can be found here: http://mediaassets.caltech.edu/gwave
7. ANTONOV AN-225 MRIYA PLANE
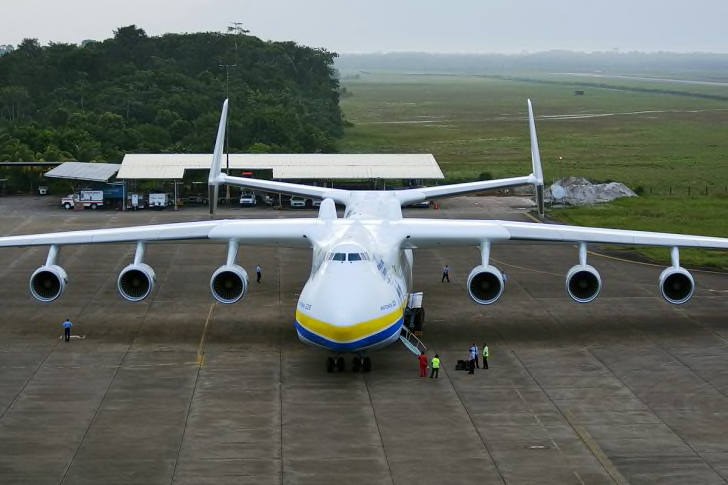
The Russians originally had a rival to the U.S. Space Shuttle program: a reusable winged spacecraft of their own called the Buran—and in the 1980s, they developed the AN-225 Mriya in order to transport it. With a wingspan the size of the Statue of Liberty, a 640-ton weight, six engines, and the ability to lift into the air nearly a half-million pounds, it’s the longest and heaviest plane ever built. Mriya first flew in 1988, and since the Buran was mothballed in 1990 after just one flight (due to the breakup of the Soviet Union rather than the plane’s capabilities), the AN-225 has only been used sparingly.
The monster plane has inspired new ideas. In 2017, Airspace Industry Corporation of China signed an agreement with Antonov, the AN-225’s manufacturer, to built a fleet of aircraft based on the AN-225’s design that would carry commercial satellites on their backs and launch them into space. Currently, virtually all satellites are launched from rockets. Meanwhile, Stratolaunch, a company overseen by Microsoft co-founder Paul Allen, is building a plane that will be wider (but not longer) than Mriya. The giant plane will carry a launch vehicle headed for low-Earth orbit.
BONUS: 10,000-YEAR CLOCK
spacer
This forward-thinking project, funded by Amazon and Blue Origin founder Jeff Bezos, focuses on reminding people about their long-term impact on the world. Instead of a traditional clock measuring hours, minutes, and seconds, the Clock of the Long Now measures times in years and centuries. The clock, which will be built inside a mountain on a plot of land in western Texas owned by Bezos, will tick once per year, with a century hand that advances just once every 100 years. The cuckoo on the clock will emerge just once per millennium. Construction began on the clock in early 2018. When this massive clock is completed—timeline unknown—it will be 500 feet high. What will be the impact of this one? Only the people of the 120th century will be able to answer that question.
ANOTHER INCREDIBLE WASTE OF TIME AND MONEY.
spacerSKB International
is our businessMore than three decades of know-how – Valuable knowledge
This is SKB International in shortSKB International helped to reduce time spans, costs and risks in national programmes in more than 20 countries since 1984. And the work continues.
We provide technology and management support for the safe disposal of spent nuclear fuel and radioactive waste.
Final repository for spent nuclear fuel
On March 16th 2011, after three decades of carrying out research, developing technology and conducting surveys, SKB applied to the authorities for permission to build a repository for spent nuclear fuel in Forsmark and an encapsulation plant in Oskarshamn. It basically concerns the final solution for the spent fuel from Swedish nuclear power plants.
spacer
Final repository for spent nuclear fuelMar 21, 2017
21 They have moved me to jealousy with that which is not God; they have provoked me to anger with their vanities: and I will move them to jealousy with those which are not a people; I will provoke them to anger with a foolish nation.
22 For a fire is kindled in mine anger, and shall burn unto the lowest hell, and shall consume the earth with her increase, and set on fire the foundations of the mountains.
23 I will heap mischiefs upon them; I will spend mine arrows upon them.
24 They shall be burnt with hunger, and devoured with burning heat, and with bitter destruction: I will also send the teeth of beasts upon them, with the poison of serpents of the dust.
25 The sword without, and terror within, shall destroy both the young man and the virgin, the suckling also with the man of gray hairs. Deuteronomy 32
![]() |
![]() |