Warning: Undefined array key "file" in /home/eyeopeningtruth/public_html/wp-includes/media.php on line 1763

RESTORED: 4/26/23
Astro=STARS. The bible says that GOD created all the Stars of the Heavens and named them. He assigned them each a task and a place. Some stars remained in one place and some he called wandering stars /planets. But even the “Wandering plantes had boundaries set by GOD. These Stars were actually also called Angels. They are entities not rocks. God made them luminous, gave them light. Just as he gave light to the Sun and a different light to the Moon. Some of these Stars/Angels left their place and came down to earth. They broke the laws of heaven.
These are knowns as the Fallen Angels. They corrupted all life on earth by mixing seed. These Fallen Angels were worshiped by the ancients as gods and demigods. They ruled over the earth until Christ came and redeemed the Earth and mankind. We have been living in the AGE OF GRACE. But God’s word says the fallen would return to Earth to wreak havoc. Those who have rejected Christ and chosen the pagan path worship there entities. They have been preparing the earth for their return.
THAT is what all this is about. Demonic spirit have been guiding and directing their path. Giving them hidden knowledge. It is not because of man’s evil that all the knowledge is alway ends up being used for evil and destruction. It is due to the evil influence of the Powers of Darkness.
Believe me when I tell you that the ELITE KNOW DEMONS ARE REAL! They also know the truth about space.
For we wrestle not against flesh and blood, but against principalities, against powers, against the rulers of the darkness of this world, against spiritual wickedness in high places. Ephesians 6:12
astro-
Entries linking to astro- *ster- (2)
“application of photography to the stars, sun, planets, etc.,” 1858, from astro- + photography.
spacer
“Astrofest La Palma” opens the doors for registration.
Posted By sheila on August 21, 2015
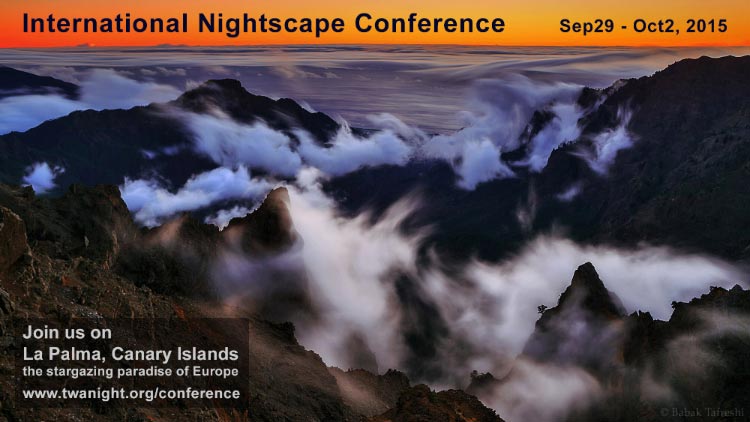
From 25th September 2015 to October 9th, the island of La Palma in the Canaries will host Astrofest, a festival of astronomy, with activities for all ages and knowledge levels. There will be an international conference for astrotourism professionals, a spectacular total eclipse of the moon, an international conference for nightscape photographers, and a night photography masterclass. You can find more details and register for all these events at the festival’s website,http://astrofestlapalma.com/
The program begins with the first International Astrotourism Conference, on September 25-27th. This is the first meeting of its kind, and it will bring together travel agents, tourist boards, scientists, environmentalists, Starlight Guides and others, to pool their experiences and reflections on the astro-tourism.
The beautiful island of La Palma is the perfect place for this fiesta because it has the best night sky in Europe: dark, clean and calm. Just ask the people who chose it as the site for the world’s biggest single mirror telescope, Gran Telescopio Canarias, along with the other 16 telescopes which make up the Observatory of the Roque de Los Muchachos. La Palma is also famous for it’s beautiful scenery in the daytime. On a clear day from the island’s summit you can see 143 km to Tenerife, and on a clear night you can see two million light years to the Andromeda galaxy.
spacer
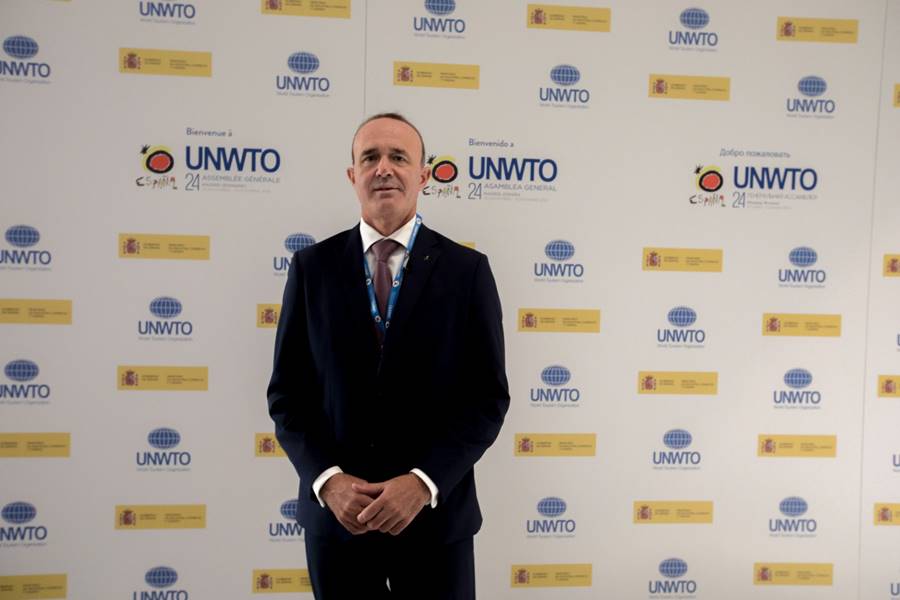
The Cabildo de La Palma, through the Ministry of Tourism, reinforces scientific and astronomical tourism by incorporating the ‘Astrofest La Palma 2022′ to the annual agenda of the World Tourism Organization (UNWTO).
This fact is the result of the meeting held by the Palma Tourism Service at the twenty-fourth General Assembly of the World Tourism Organization held the first days of December in Madrid.
The counselor, Raúl Camacho, highlights the importance of the tourism sector and astrotourism in the island economy, both in terms of social development and job creation, “which makes it an essential engine for recovery that, now more than never, is it necessary to boost on the island to counteract the impact that the volcano’s eruption is having ”.
After the forty-third plenary session of the Affiliate Members of the UNWTO, it has been concluded that for the work program of 2022 the aim is to give relevance to scientific tourism since the combination of science and tourism on the horizon of the tourism industry provides opportunities for developed destinations and makes them more sustainable.
This is why they are constantly pushing tourism throughout thi volcanic event. That is also why the spend so much time and money investing in all the scientific documentation, sample collecting, technical testing and evaluating of eveery aspect of the event and promototing it through pictures, videos an social media.
Raúl Camacho also assures that ‘Astrofest La Palma 2022′ “will be key to promoting the island as a differentiated astrotourism destination, thanks to the quality of its sky, an attraction that will also be reinforced in the coming months with the opening of the Center Visitors to the Roque de los Muchachos ”.
In this way, he underlines in a note, “we will increase the profile of La Palma as an island of international reference in astronomy, astrophysics and astrotourism, while raising awareness about astrotourism as a sustainable tourism product for destinations around the world.”
![]() Ministerio de la Presidencia
![]() @M_Presidencia
The Government of Spain foresees that by the end of the year it will have mobilized 240M € for the reconstruction of the island of #LaPalma in coordination with all the administrations. LINK TO VIDEO: https://twitter.com/i/status/1468614327045394441 |
The UNWTO scientific tourism working group agreed to work in 2022 on a guide on the creation of the astrotourism product that will serve as a manual of good practices and development of its capacities for tourism public administrations that want to diversify their offer.
In addition, assess all the direct and indirect benefits derived from the protection of the sky such as environmental, cultural, scientific, biodiversity, health, quality of life, and socioeconomic, through astrotourism.
spacer\
La Palma is known by many names
The Star Spangled Sky |
The Sky of the Stars |
The Stars Island | |
The Dark Island | |
La Palma Europe’s Hawaii |
The beautiful island |
cielo de as estrellas / Star Sky
Imagine all the stars in the galaxy spinning in the sky. The bluish-white nuclei of the galaxy’s young stars are surrounded by the yellow octopus arms of their older sisters. On one side, an almost invisible red column of gas snakes away from the starry whirlpool, turns in the middle of the sky, and begins to approach … you.
I see those images in the Volcanic event at La Palma. The lava octopus, the red gassy snakes rising up from the ground and the whirlpool created by the Stromboli explosions of lava dancing around the mouth of cone as the reach into the sky.
spacer
La Palma The Star Spangled Sky
La Palma has also been described as a miniature continent. The island shows a thousand facets, not only during the day, but also at night. Every night, the sky above the small Canarian Island turns into a glittering starry firmament.
We all know the Milky Way, but have you ever seen it with your naked eye? The spangled sky of La Palma is truly impressive … you shouldn’t miss this experience. On the island’s highest mountain, the Roque de los Muchachos (2.426 m), this display of nature turns into a true spectacle, for here, the stars seem to be within the viewer’s grasp.
Let us tell you why.
5 reasons why La Palma has one of the most beautiful starry skies of the world
1. Law against light pollution
Yes, this law really exists and regulates the luminous intensity and the alignment of exterior lighting. The darker the night, the better we can see the spangled sky.
2. The sky is clear and pure
The sky above La Palma is amongst the clearest and purest of the world and offers a clear view, almost like in space.
3. Flyover ban
It is illegal to fly over the island with passenger planes which protects the sky from being polluted.
4. Science; Scientists
On the Roque de los Muchachos, one of the world’s largest telescope arrays is located. Many scientific facilities are located on La Palma because of the uniqueness of its spangled sky. Even the prominent scientist Stephen Hawking came to look at it a few years ago.
5. Almost no industry
La Palma has no industry to speak of and no big cities. Here, the small island amidst the Atlantic Ocean scores doubly.
All this makes La Palma a special place for discovering the stars. See for yourself!

Marina Manganaro
Yes but with more telescopes (In the image one of the @MAGICtelescopes
I am working with, on the Canary Island of #LaPalma, Credit: Daniel Lopez (@IAC_Astrofisica)
Aug 23, 2015
Stars Island La Palma | The Sky (Starlight Reserve)
THE SKY (STARLIGHT RESERVE)
An unpolluted night sky that allows the enjoyment and contemplation of the firmament should be considered an inalienable right equivalent to all other socio-cultural and environmental rights …
La Palma was one of the first places in the world to apply the Sky Law: in 1988 a specific law was passed designed to protect the quality of the night sky for the purpose of astrophysical observation, which was a giant step forward in defence of the sky on a world-wide scale. This law protects La Palma from light, atmospheric and radio-electrical pollution, as well as preventing interference from aviation routes. (Anythig that might interfere with their wave experiments or maneuvers)
Study of measuring light pollution made by Antonio González (Cielos-LaPalma.com) for the Cabildo Insular de La Palma (The Island’s government) at viewpoints and places of astronomical interest. Measurements made by SQM (Sky Quality Meter). The study period was from March to April 2013. The Visual Magnitude Limit of a person with standard vision, in a dark place, is 6, which is an indication of good sky quality. If it is less, this is due to light pollution. However the Sky Quality Meter (SQM) provides a logarithmic scale of magnitude values per squared arcsec. It gives values ranging from 16 to 22, the latter being the maximum for dark skies free of light pollution.
Thanks to its unique astronomical conditions of clearness and darkness, in April 2007 La Palma was declared Starlight Tourist Destination and Starlight Reserve within The International Conference in Defense of Quality of the Night Sky and the Right to Observe Stars.
Moreover, the amazing sky of La Palma is protected by the Law 31/1988, also known as The Sky Law, which covers four major aspects:
- Light pollution
Public lighting installations have to comply with basic standards in order to preserve the darkness of night and enable the enjoyment of the starry sky, as well as many other benefits.
- Radioelectric pollution
Radiocommunication stations have to operate within a maximum limit of power flux density, in order to prevent interferences between the electromagnetic radiation and the telescopes of the Observatory.
- Atmospheric pollution
Industries and polluting activities are not permitted above 1,500 meters of altitude.
- Flights routes
Air traffic is regulated over the Observatory in order to prevent turbulence caused by aircrafts.
Why La Palma is the Island of the Stars in Canary Islands?
La Palma: the island of the stars
A timelapse of a stunning night sky

The Dark Island from Nicholas Buer on Vimeo.
This film was shot over 11 nights in March on La Palma, Canary Islands.
La Palma is often called ‘Europes’ Hawaii’ as it has all the ingredients for a perfect night sky cocktail; altitude, dry air and a lack of light pollution. These elements when combined make for a stunning night sky. Because of it’s clean air and clear skies many of the worlds top observatories have facilities on top of the island.
The main goal of this film was to capture the Milky Way rising from the horizon at a low angle across the sky. There are two main factors that affect the position of the Milky Way; latitude and time of year.
Latitude is your distance either North or South from the Earth’s equator. In the Northern Hemisphere the closer you are to the equator the higher the central core of the Milky Way will rise into the sky. La Palma is positioned at 28˚ North, which is a good latitude to photograph the central core of the Milky Way.
Time of year affects the angle in which the Milky Ways appears in the sky. The general consensus is that the Milky Way season starts in March and ends in October. In March the angle in which the Milky Way rises in the Northern Hemisphere is at its’ most acute. As Spring turns into Summer the Milky Ways angle increases tilting until it is upright through the months of June to August, then falls away back on itself slightly through September to November until the core disappears from view through the Winter months. (The Timing of the event /the Volcano)
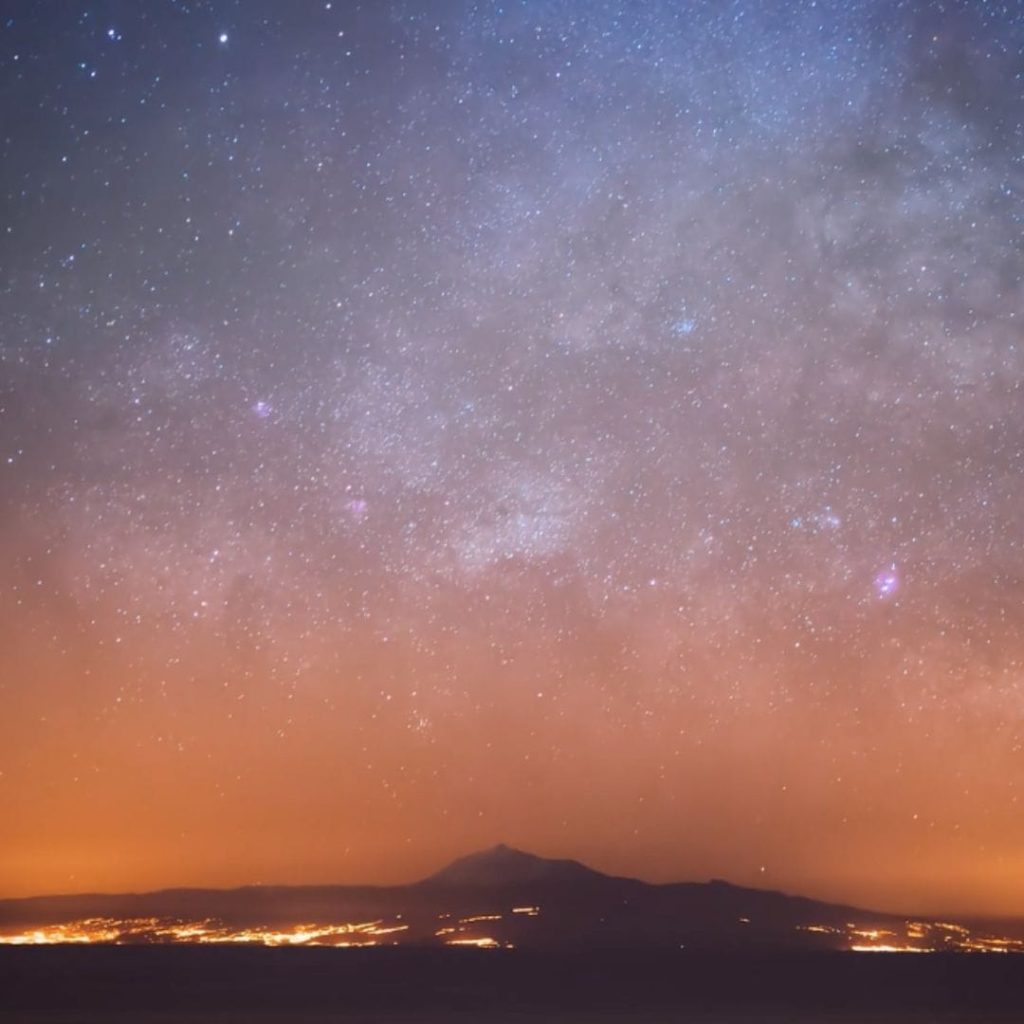
Nov 20, 2021



Gerardus Mercator
If you have ever seen a map of the world in a classroom or in an atlas, chances are you have seen a version of a “Mercator projection.” You may not, however, be familiar with its creator, Gerardus Mercator.
Mercator World Map
Geradus Mercator’s world maps flattened the spherical planet to make it easier to display. Displays of the landmasses are not necessarily proportional to their actual size, especially toward the poles. Despite these distortions, his maps are still in heavy use. Though Mercator is best known for his cylindrical maps, he created various map types, like this spherical map.
IMAGE BY MARY EVANS/SCIENCE SOURCE
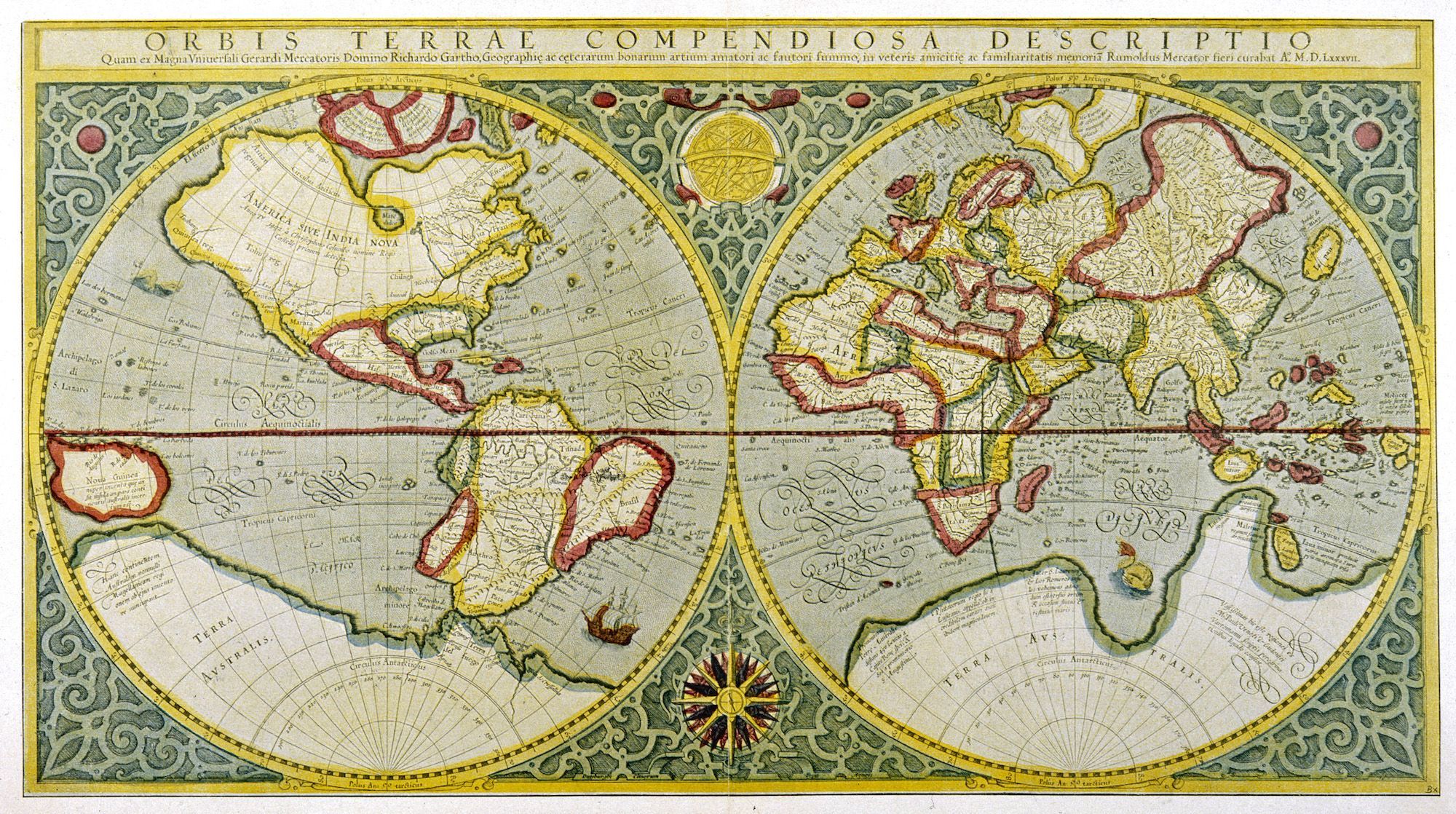
Mercator was born in Flanders (located in modern-day Belgium) in 1512. The son of a cobbler, Mercator grew up in a poor family. He graduated from the University of Louvain in 1532, where he studied mathematics, geography, and astronomy. After graduating, Mercator developed his skills as an engraver, calligrapher, and geographer, and then began making globes and scientific instruments. As his reputation grew, Mercator published several maps of places around the world. These included an early version of his world map, which showed the globe as a heart-shaped projection.
He continued studying the sciences and making maps and instruments for wealthy, and sometimes high-profile, clients. But in 1544, Mercator was arrested under suspicion of heresy; the traveling he did for research had made church officials wary. After spending a few months in prison, he was released and continued his studies.
In 1569, Mercator published his epic world map. This map, with its Mercator projection, was designed to help sailors navigate around the globe. They could use latitude and longitude lines to plot a straight route. Mercator’s projection laid out the globe as a flattened version of a cylinder. All the latitude and longitude lines intersected at 90-degree angles. Because the projection was intended to be a reference for navigation and not land geography, the landmasses on the map are not necessarily proportional to their actual size; at higher latitudes, landmasses appear larger than their actual size. Despite these distortions, Mercator’s projection is still heavily used today.
In addition to publishing his famous projection, Mercator was the pioneer of another geographical tool we use to this day. He coined the term “atlas” (named after the Greek mythological figure who held the world on his shoulders) to describe a collection of maps. Mercator continued his cartography work for the rest of his life, publishing parts of his atlas until his death in 1594.
Geradus Mercator’s world maps flattened the spherical planet to make it easier to display. Displays of the landmasses are not necessarily proportional to their actual size, especially toward the poles. Despite these distortions, his maps are still in heavy use. Though Mercator is best known for his cylindrical maps, he created various map types, like this spherical map.
Image by Mary Evans/Science Source
Azimuthal Equidistant “Air Map” of 1945 proves Flat Earth
A compass tells the direction in which the ship is going or the direction of any other object.
An azimuth mirror is used in conjunction with a compass.
This device enables the operator to measure bearings of celestial and terrestrial objects.
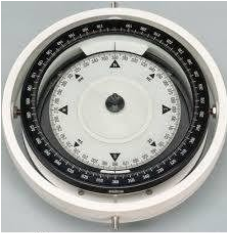
Purpose of Azimuth mirrors (Azimuth Circle)
Azimuth mirror is used for taking the bearings of terrestrial and celestial objects.
By the use of mirrors or lenses or prisms or combination of all these, the equipment allows both, the readings of the compass card, and the object to be seen at the same time and in the same direction.
Thus the bearing of the object can be read out.
Azimuth mirror is therefore portable equipment which is placed over a magnetic or gyro compass for measuring bearings of terrestrial and celestial objects.
![]() |
![]() |
Sight Vanes (Near vane & far vane) |
Levels Indicate if the ring is level with the horizon. NOTE: Bearings read when the ring is not level are inaccurate. |
![]() |
![]() |
Allow the observer to take bearings of objects by aligning the two vanes to the object.
The near vane contains a peep sight while the far vane contains a vertical wire. The far vane is mounted on top of a housing that contains a reflective mirror inside enabling the observer to read the bearing from the reflected portion of the compass card. |
Concave mirror
Reflects the sun’s rays onto the prism housing on the other side of the ring when the observer is taking an azimuth of the sun. |
![]() |
![]() |
Reflector vane Allow the observer to observe azimuths of celestial bodies (stars and planets) at various altitudes by picking up their reflection in the black mirror. When the body is observed, its reflection appears behind the vertical wire in the far vane. | Prism Housing Directs the beam of light from the concave mirror downward in a narrow beam onto the compass card enabling the observer to read the azimuth to the sun. |
In a matrix, which is termed “The Circle of Mirrors”, we see the Letter M holding a key “center position”.
The Circle of Mirrors is derived from placing the symmetrical letters of the Alphabet into their “Pi positions”.
The Letters M and W are 13 and 23 respective. 13+23 = 36. The X to the right of the W is 10. 36 * 10 = 360 degrees of a circle. The V to the left of the W is 5. 360+5 is 365 days to a year.
The “I”, which shares a value (phonetic and mathematic) with Y, is in a “leap” or “elevated row”, which may then be added and termed as a “leap year”, for I is 1 and in a leap year we must add one day to bring the Solar calendar back into balance. Source: “Savior Gods”, The Letter Y and the Circle of Rhymes
Science/Alchemy/Witchcraft changed times, seasons, laws and our understanding of the Cosmos.
cer
La Palma
CTA’s site in the northern hemisphere
The Cherenkov Telescope Array (CTA) is the next generation ground-based observatory for gamma-ray astronomy at very-high energies. With more than 100 telescopes located in the northern and southern hemispheres, CTA will be the world’s largest and most sensitive high-energy gamma-ray observatory.
Northern Hemisphere Array
CTA’s northern hemisphere or CTA-North site is located on the existing site of the Instituto de Astrofisica de Canarias’ (IAC’s) Observatorio del Roque de los Muchachos in Villa de Garafia on the island of La Palma, the fifth largest island in the Canary Islands. At 2,200 metres in altitude and nestled on a plateau below the rim of an extinct volcanic crater, the site currently hosts an operating gamma-ray observatory, the Major Atmospheric Gamma Ray Imaging
Cherenkov (MAGIC) telescopes, as well as a wide variety of optical telescopes of various sizes.
spacer
The Webb Space Telescope Will Rewrite Cosmic History. If It Works.
To look back in time at the cosmos’s infancy and witness the first stars flicker on, you must first grind a mirror as big as a house. Its surface must be so smooth that, if the mirror were the scale of a continent, it would feature no hill or valley greater than ankle height.

Natalie Wolchover
‘s inshallah (+skyward glance): “Please work.”
Inshallah
In sha’Allah (/ɪnˈʃælə/; Arabic: إِنْ شَاءَ ٱللَّٰهُ, ʾIn shāʾ Allāh Arabic pronunciation: [ʔin ʃaː.ʔa‿ɫ.ɫaːh]), also spelled In shaa Allah and Inshallah is an Arabic language expression meaning “if God wills” or “God willing

See more pictures on our Flickr page.
In early August, NASA’s Fermi Gamma-ray Space Telescope entered the second half of its intended 10 year observation period, which will end in 2018. So far, the mission has not experienced technological complications and has revealed a lot about our universe by providing images of the sky in gamma rays. The Large Area Telescope (LAT) has been crucial in this process, scanning the sky every three hours and creating increasingly detailed pictures of the visible universe in gamma rays. The probe’s gamma-ray burst monitor instrument provides images of the entire sky with the exception of the part blocked by Earth.
Ultimately, This view provides the best chance of catching a glimpse of more gamma-ray bursts, solar flares, and other high-energy activity.

The telescope allows us to get a glimpse of the universe in forms of light that are not visible to our senses and in doing so, illuminates phenomena that were previously invisible to us.
For example, in 2010 bubble-like structures were detected by the Fermi telescope that stretched over more than 25,000 light-years above and below the plane of the Milky Way. Astronomers have contended that these strange bubbles could have resulted from high energy outbursts from the supermassive black hole at the center of the Milky Way galaxy. Scientists remain optimistic that the Fermi telescope and gamma-ray images will continue to provide more clues to understanding our solar system, our galaxy, and the nature of the universe.
Array Coordinates:
Longitude: 17º 53′ 31.218″ West
Latitude: 28º 45′ 43.7904″ North
While the southern hemisphere array will span the entire energy range of CTA, covering gamma-ray energies from 20 GeV to more than 300 TeV, the northern hemisphere array will be more limited in size and will focus on the low- and mid-energy ranges from 20 GeV to 20 TeV. For this reason, the northern hemisphere site will not host any Small-Sized Telescopes, which are tuned to capture the highest-energy gamma rays. The plan is for the site to host four Large-Sized Telescopes to capture the low-energy sensitivity of CTA and 15 Medium-Sized Telescopes to cover CTA’s core energy range.

»Four LSTs will be arranged at the centre of both the northern and southern hemisphere arrays to cover the unique low energy sensitivity of CTA between 20 and 150 GeV.«
The Large-Sized Telescope (LST) project team consists of more than 100 scientists from ten countries: Brazil, Croatia, France, Germany, India, Italy, Japan, Poland, Spain and Sweden. Because gamma rays with low energies produce a small amount of Cherenkov light, telescopes with large mirrors are required to capture the images. Four LSTs will be arranged at the centre of both the northern and the southern hemisphere arrays to cover the unique low energy sensitivity of CTA between 20 and 150 GeV. The LSTs will also have a very good sensitivity up to energies of several TeV, which is, however, covered by Medium-Sized Telescopes (MSTs) more efficiently.
The LST is an alt-azimuth telescope. It has a 23 m diameter parabolic reflective surface, which is supported by a tubular structure made of reinforced carbon fibre and steel tubes. A reflective surface of 400 m2 collects and focuses the Cherenkov light into the camera, where photomultiplier tubes convert the light in electrical signals that can be processed by dedicated electronics. Although the LST will stand 45 m tall and weigh around 100 tonnes, it will be extremely nimble, with the goal to be able to re-position within 20 seconds.
Link: Large-Sized Telescope
![]() |
LST Specifications |

- spacer
- An illustrative schematic of the telescope with the main assemblies:

Both the re-positioning speed and the low energy threshold provided by the LSTs are critical for CTA studies of galactic transient, high red-shift active galactic nuclei and gamma ray bursts. The LSTs will expand the science reach to cosmological distances and fainter sources with soft energy spectra.
»The LST camera has a total field of view of about 4.3 degrees and has been designed for maximum compactness and low weight, cost and power consumption while providing optimal performance at low energies.«
LST Camera
The LST Camera shares many elements with the NectarCAM for the MSTs. It has a weight of less than two tonnes, a total number of channels of 1,855 divided into 265 photomultiplier tube (PMT) modules that are easy to access and maintain. PMTs with a peak quantum efficiency of 42 percent are used as photosensors to convert the light into electrical signals. To maximize the light throughput, each photosensor is equipped with an optical light concentrator, optimized for the field of view and geometry of the photosensor.
The camera has a total field of view of about 4.3 degrees and has been designed for maximum compactness and low weight, cost and power consumption while providing optimal performance at low energies. Each pixel incorporates a photosensor and the corresponding readout electronics. These electronics are based on the Domino Ring Sampler Version 4 (DRS4) chip, which is developed at the Paul Scherrer Institute in Switzerland and currently used by several experiments; among them the MAGIC Cherenkov telescopes.
The camera trigger strategy is based on the shower topology and the temporal evolution of the Cherenkov signal produced in the camera. The analogue signals from the photosensors are conditioned and processed by dedicated algorithms that look for extremely short but compact light flashes. Furthermore, the LST cameras are interconnected in order to form an on-line coincidence trigger among the telescopes, which helps suppress accidental triggers by up to a factor of 100.
LST Prototype
The construction of the LST prototype, LST-1, was completed in October 2018 in La Palma, Canary Islands, Spain, on the site of the Observatorio del Roque de los Muchachos. The prototype is foreseen to become the first LST telescope of CTA, and, in fact, the first telescope on a CTA site, to be operated by the CTA Observatory (CTAO). As any other technical delivery in the large, multinational CTA project, the LST-1 will need to undergo a critical design review to verify that the design complies with CTA science goals, operational needs, safety standards, etc. before it is formally accepted by CTAO.
spacer
Sep 13, 2010
the robotic Liverpool telescope ( http://www.iac.es/eno.php?op1=2&op2=3… ) and
the Isac Newton Telescope ( http://www.iac.es/eno.php?op1=2&op2=3… ).
–Venus (it is a planet indeed, but looks like a very bright star) can be seen setting above the horizon at 00:48 and 01:00 (Mercury is also visible to the lower right at 01:02). -Several satellites, but also some meteors can be seen (e.g., at 02:35). -Shadow cast by the mountain at sunrise can be seen on the clouds at 03:43. -The last morning dawn scene was shot from the Roque de los Muchachos, which is the highest point of La Palma, 2423 m ASL. Created from ~5000 individual photographs taken with a Canon EOS 7D + a Canon EF-S 15-85mm f/3.5-5.6 IS USM lens (usually set at 15 mm and wide open for the night-time shots). Original cuts were rendered in Photoshop CS4, then the movie was cut together in iMovie (this degraded the final quality slightly during compression). Music: Utopia by Goldfrapp, © 2010 Mute Records Ltd. Inspired by Daniel López ( http://www.tierrayestrellas.com/cielo… ).
Stars Island La Palma | Cielos La Palm
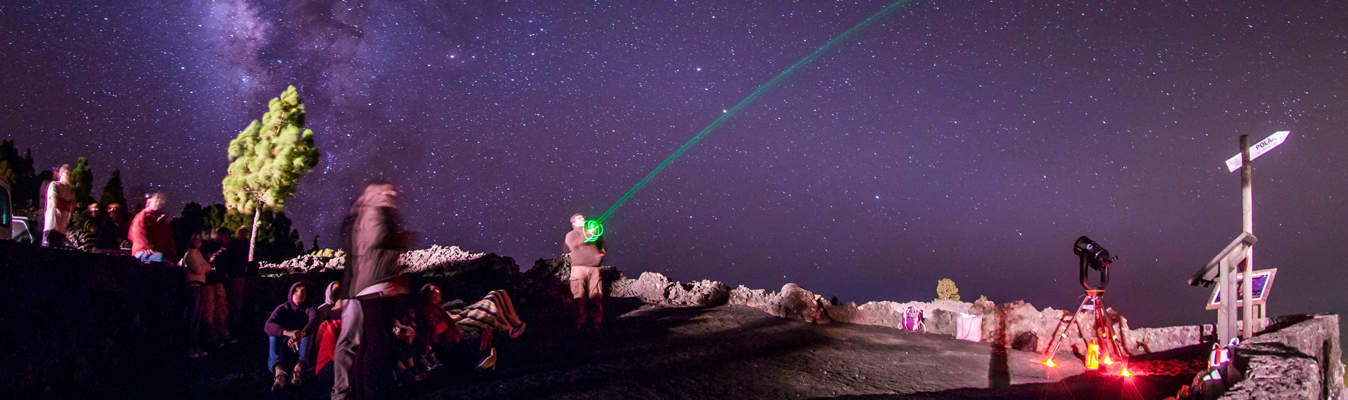
LA PALMA SKY
Cielos La Palma (lapalma-sky.com) is an Astronomy-related brand that also offers activities from other disciplines too, such as pre-history, tales and legends, mythology, caving and hiking and active leisure activities. The company is headed up by Antonio González, who has over 25 years experience in astronomy dissemination. He is also a Starlight Guide and an expert in Astro-Tourism. He invites you to discover the island of La Palma in a different fashion.
-
- Equipment: Telescopes Celestron C11, C9.25, C8 and C6, Meade ETX125 and Dobson 8″, APO 66mm, binoculars 11×80, 20×80 and 10×50, APO Giant Binocular 100mm, 2 Telescopes H-alfa Lunt and solars filters, cameras 5Dmii, modified cameras, 6D and 650D, and timelapses equipement
La Palma conviviendo con el Universo (La Palma Living with the Universe)
spacer
The Observatory
Because La Palma has such amazing skies, the professional astronomers have moved in. So far there are sixteen telescopes at the observatory of the Roque de Los Muchachos.
The northern end of the Roque de Los Muchachos. From left to right, the ATC (half hidden by the bushes), WHT, DOT, LT, SST, Mercator (behind the SST), INT and JKT.
|
Immediately adjacent to the next tower We can see the Swedish 1-m Solar Telescope (SST), the largest in Europe and number one in the world when it comes to high spatial resolution. The last two white domes belong to the 2.54m Isaac Newton Telescope (INT) and the 1m Jacobus Kapteyn Telescope (JKT).” Atmospheric Optics
|
Astronomers describe telescopes by the diameter of the main mirror or lens. From biggest to smallest, the telescopes on La Palma are:
MAGIC: Major Atmospheric Gamma-ray Imaging Cherenkov telescope. Two 17m mirrors which work together like binoculars, to observe gamma rays. Run by a consortium of over twenty institutions from nine countries.
With a reflector diameter of 17 meters each, the two MAGIC telescopes are the most sensitive Cherenkov telescopes in the world, especially in the energy range below 200 gigaelectronvolts (GeV). Their line of sight is directed at objects that emit gamma rays ranging from 30 GeV to 100 TeV (teraelectronvolts). This means that MAGIC can cover an enormous energy spectrum.
CTA-NORTH SITE: OUR NORTHERN EYE ON THE HIGH ENERGY UNIVERSE
The twin telescopes are located 2,200 meters above sea level on the Canary Island of La Palma where the clear skies and lack of light pollution make for optimal observing conditions. The Max Planck Institute for Physics (MPP) leads the international collaboration of about 165 astrophysicists from 24 research institutions in eleven countries. Together, they are responsible for the construction, operation and maintenance of the telescopes. MAGIC allows astrophysicists to obtain first class data for gaining scientific insights into enigmatic objects and the most violent processes in the universe.
The MAGIC telescopes have been in operation since 2003 and 2009 respectively. The MPP played a major role in the development and construction of their mechanical structure, imaging cameras and calibration system.
Since the outset, MAGIC has delivered many valuable scientific discoveries.
It’s one thing to see all those white domes lined up across the side of the mountain. It is another to see the actual telescopes that are inside them. I tried to find photos of as many as I could. There seems to be scertain ones that they do not want anyone to see.
Grantecan: Grand Telescopio Canaria the big Canarian Telescope.
GranTeCan is the biggest optical telescope in the world, and it’s hard to convey the sheer size of it in a photograph. The telescope itself weighs 485 tonnes. You could fit an tennis court inside the dome, and the top of the dome is 41 m above the ground. But you can’t see any of that in a photograph.
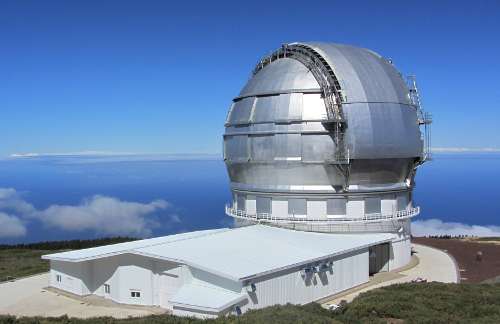
This is the biggest and newest optical telescope in the world, with a 10.4 m segmented mirror. Run by the Spanish Instituo de Astrofisica de Canarias.
WHT: The William Herschel Telescope
The William Herschel Telescope was for many years the biggest and best optical telescope in Europe (until GranTeCan opened in 2009). The main mirror is 4.2 m across (165″, or 13′ 9″) which astronomers call “a good light bucket”. It’s rather old as world-class telescopes go, since it opened in 1987, but it still produced excellent science. In fact data from the WHT has been used for about 1,500 scientific papers. It helps that it’s been fitted with adaptive optics.

This is when you use some starlight to measure the air turbulence, and then deform a special, flexible mirror to compensate for that turbulence. It’s rather like using glasses to correct for the shape of your eyeball, but these glasses change shape 100 times a second.
This only works if you have a bright star handy, in order to measure the turbulence in the first place. Some parts of the sky have far more stars than others, so the WHT has a laser, which can be used to create an artificial star. To the best of my knowledge, it’s the only one working in Europe (although GranTeCan will have one too.)
The telescope’s named after Frederick William Herschel, who was born in Germany but emigrated to England. He started life as a musician, but music lead to mathematics and then to astronomy. He’s best known for discovering the planet Uranus, but he also measured the height of the mountains on the moon, discovered double stars, catalogued loads of nebulas, found two of Saturn’s moons and two of Uranus’s moons, and was the first to realise that the solar system is moving around the galaxy. Oh, and he discovered infra-red radiation. Pretty impressive for someone who didn’t really get started on astronomy until his mid-forties. (Obviously there’s hope for me yet.)
The William Herschel Telescope, with a 4.2 m mirror and many instruments to observe in visible light and infrared. Run by the Isaac Newton Group, a consortium of the UK, Netherlands and Spain.
pt5m – a 0.5 m robotic telescope on La Palma
pt5m2 is a 0.5 m robotic telescope located on the roof of the William Herschel Telescope (WHT) on La Palma (Fig. 1) and is hosted by the Isaac Newton Group of Telescopes (ING). It functions as an astrophysics research facility, an atmospheric turbulence profiler, and a teaching resource. pt5m has already been used to study pulsating stars, transiting exoplanets, Solar system objects, interacting binaries (Littlefair et al. 2013; Kupfer et al. 2014; Campbell et al. 2015), and transients (Gandhi et al. 2014; Hardy et al. 2015).
Brief History of pt5m
pt5m was first developed as an atmospheric turbulence profiling (SLODAR) facility (Wilson 2002) and was originally installed at the South African Astronomical Observatory (Catala et al. 2013). The telescope was then moved to La Palma in 2010 to support the CANARY adaptive optics demonstrator (Morris et al. 2009). For this reason, it was sited as close as possible to the WHT, to sample the turbulence in approximately the same beam as the WHT.
In order to fully utilize the telescope when not being used for SLODAR observations, we decided to add a science imager in 2011. We recognized that even a small telescope located at such an excellent site could provide a powerful tool for time-domain astronomy. The science CCD and integrated filter wheel (see Section 3.2) were originally mounted on a linear slide to move the science camera in and out of the light path of the SLODAR optics. By 2012 we had implemented the robotic mode (Section 4) and started using pt5m on a routine basis for time-resolved and transient astronomy. In 2014, the original 0.5 m Orion Optics Modified Dall–Kirkham telescope was replaced with a new Ritchey–Chretien telescope (Section 3.3). This refurbishment was made to improve throughput and image quality, which were not a high priority for the original SLODAR studies. At the same time the slide mechanism was removed and replaced with a flip mirror that allows light to be diverted to either one of two optical ports. One of these ports hosts the science camera and the other hosts the atmospheric turbulence profiler (see Fig. 2 and Section 3.3).
TNG: Telescopio Nazionale Galileo . The Italian national telescope has a 3.5 m mirror.
![]() |
![]() |
INT: The Isaac Newton Telescope has a 2.5 m mirror, and is also run by the Isaac Newton Group. The Isaac Newton Group of Telescopes (ING) operates the 4.2 metre William Herschel Telescope (WHT) and the 2.5 metre Isaac Newton Telescope (INT) at the Roque de los Muchachos Observatory, La Palma, Spain.
NOT: Nordic Optical Telescope also has a 2.5 m mirror, and is run by a consortium of Denmark, Finland, Iceland, Norway and Sweden.
![]() |
|
Nordic Optical Telescope | Instituto de Astrofísica de …iac.es
588 × 516
|
Nordic Optical Telescope – Wikipediaen.wikipedia.org
500 × 375
|
![]() |
![]() |
Mercator: The Mercator telescope has a 1.2 m diameter, and is run by Leuven University in Belgium and the Observatory of the University of Geneva.
![]() |
![]() |
SQT: the brand new SuperWASP Qatar Follow-up telescope has a 1 m mirror, and as the name suggests, it isused to confirm whether stars observed by SuperWASP really do have planets orbiting them. SuperWASP consists of two robotic observatories that operate continuously all year around, allowing us to cover both hemispheres of the sky. The first, SuperWASP-North is located on the island of La Palma amongst the Isaac Newton Group of telescopes (ING). The second, SuperWASP-South is located at the site of the South African Astronomical Observatory (SAAO), just outside Sutherland, South Africa. The observatories each consist of eight wide-angle cameras that simultaneously monitor the sky for planetary transit events. A transit occurs when a planet passes in front of its parent star temporarily blocking some of the light from it (see the How it works section). The eight wide-angle cameras allow us to monitor millions of stars simultaneously enabling us to detect the rare transit events. Details of the planets discovered by the WASP project can be found here.
JKT: Jacobus Kapteyn Telescope has a 1m mirror. It opened in 1984, but is no longer in general use.
The Jacobus Kapteyn telescope weighs about 40 tonnes and has a 1 m mirror on an equatorial mount. It was finished in 1984, in time for the inauguration of the observatory and it was in constant use until 2003. Since then it was used for short periods, until it was handed over to SARA, the Southeastern Association for Research in Astronomy (a consortium of 12 US universities plus the IAC). They plan to run it robotically.
NSF to Refurbish La Palma Telescope – Texas A&M University …
SST: The Swedish Solar Tower observes the sun, as the name suggests. It has a 1 m lens.
The Swedish 1-m Solar Telescope is a refracting solar telescope at Roque de los Muchachos Observatory, La Palma in the Canary Islands. It is run by the Institute for Solar Physics of Stockholm University. The primary element is a single fused silica lens, making it the second largest optical refracting telescope in use in the world. The 110-cm lens has a clear aperture diameter of 98 cm. The SST is most often used as a Schupmann telescope, thereby correcting the chromatic aberrations of the singlet primary.
The SST is a vacuum telescope, meaning that it is evacuated internally to avoid disruption of the image from air inside. This is a particular problem with solar telescopes because of the heating from the large amounts of light collected being passed on to any air causing image degradation.
As of 2005 the SST has produced the highest resolution images on the Sun of any telescope. This is largely thanks to its adaptive optics system, which was upgraded to an 85-electrode monomorph deformable mirror from CILAS in 2013.
There are two modes of operation, selected by switching the beam from one optical table to another. One mode is a spectrograph mode, using the TRIPPEL spectrograph. The other mode is an imaging mode, where the beam is split up in a red and a blue part by a 500-nm dichroic beamsplitter. Both beams have dual Fabry-Pérot-based tunable filters, CRISP in the red and CHROMIS in the blue. The image data are usually compensated for residual wavefront aberrations by use of the MOMFBD image reconstruction method.
The SST superseded the SVST – the Swedish Vacuum Solar Telescope – which was 47.5 cm in diameter.
KVA-60: A 60 cm reflector owned by Sweden. The oldest telescope still working at the observatory.
DOT: The Dutch Open Telescope also observes the sun, this time with a 0.45 m lens.
ATC: The Automatic Transit Circle (0.18 m) refracting telescope, used for measuring star positions. It was built in Copenhagen in 1956 and started work on La Palma in 1984. the small white house is the home for the Automatic Transit Circle (ATC), an old meridian circle built by Grubb-Parsons in 1950 but completely refurbished and automated in the 1970’s by the Copenhagen University Observatory (CUO).
SuperWASP has 8 lenses, each 11 cm in diameter to search for extrasolar planets – planets outside our solar system.
CILBO: 25mm video camera for tracking meteors.
You can visit the observatory for free, but you have to sign up in advance at http://orm.astrocamp.es/index_en.html
The observatory is at 2400 m (7,900 ft) and there is about 25% less oxygen than at sea-level. This is fine for most people until they try to climb stairs quickly, but you probably shouldn’t come if you have anaemia or a serious lung or heart problem, and think carefully about bringing babies or the very old. If in doubt, talk to a doctor. Please consult http://www.iac.es/adjuntos/orm/recommendations-visitors.pdf And bring your own sun cream, water, and food (especially if you’re diabetic).
spacer
Starlit Skies
Posted By sheila on August 29, 2019
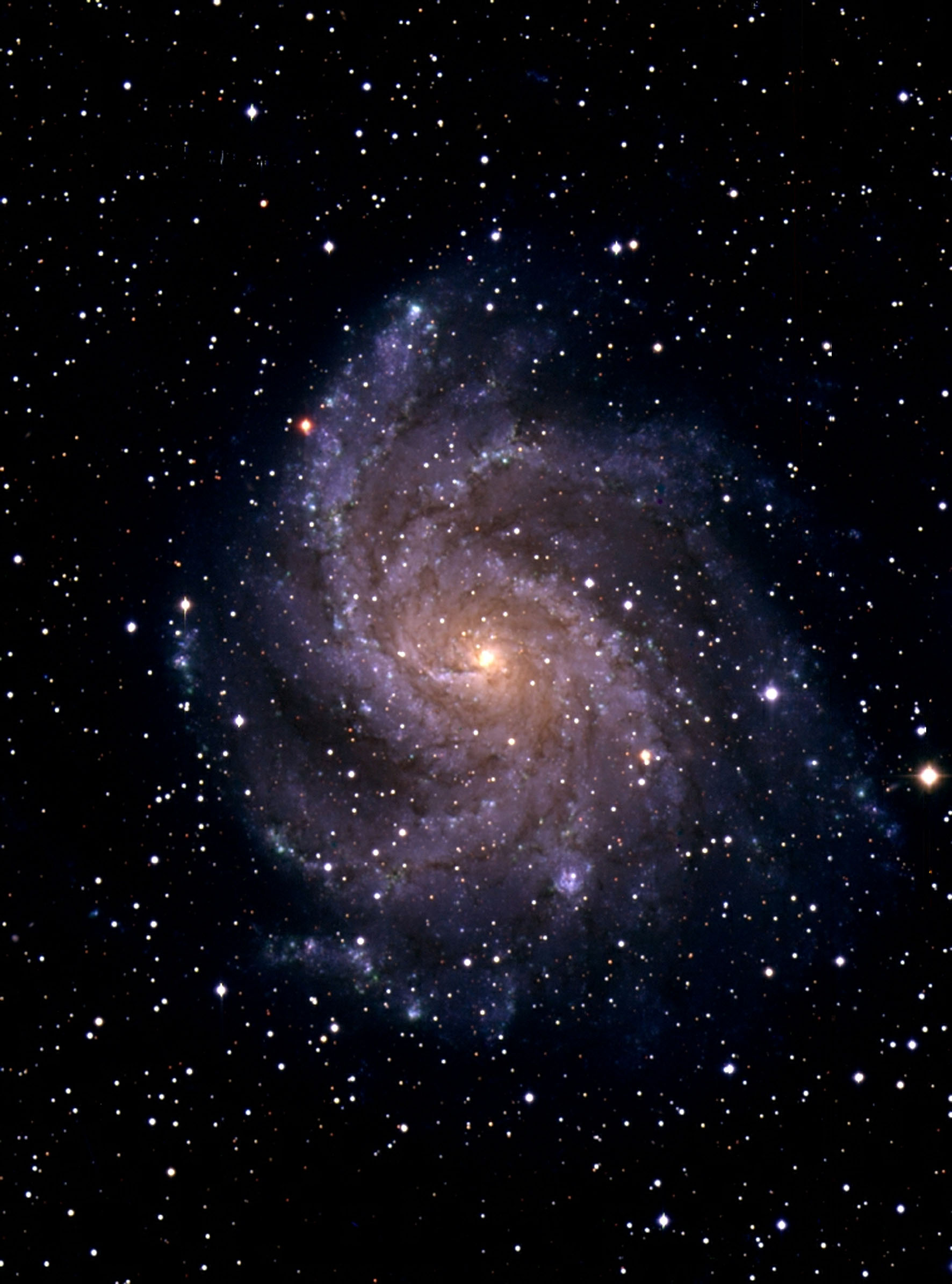
.acer
Atmospheric Extinction at the Roque de los Muchachos Observatory,
La Palma
D L King (RGO)
6 September 1985
Atmospheric extinction at the Roque de Los Muchachos Observatory
Introduction
The wavelength dependence of the atmospheric extinction at the Roque de los Muchachos Observatory is currently in the process of being measured. Until these data become available, a satisfactory approach for many applications is to correct spectroscopic and photometric observations using a theoretical extinction curve, calculated as suggested by Hayes and Latham (1975). Comparisons between calculated and observed mean extinction coefficients for Lick and Cerro Tololo observatories appear to suggest that the calculated extinction curve is
probably accurate to within a few hundredths of a magnitude. This is also borne out by available data for La Palma (see below).
This note gives such a theoretical extinction curve, appropriate to the altitude of the Roque de los Muchachos observatory and covering the spectral range 3000-11000 A. The data are presented in graphical and tabular form.
Furthermore, this extinction curve is available to SPICA Users in the VAX file:
RGVAD::LDRUGDIR:LAPALMA.EXT
Calculated Extinction Curve
The three main contributions to extinction by the Earth’s atmosphere of relevance to ground-based astronomy are Rayleigh scattering by air molecules, molecular absorption and aerosol scattering. The method outlined by Hayes and Latham (1975) gives the mean extinction for an aerosol-free atmosphere, as follows:
1) Rayleigh scattering
The wavelength (l in mm) and altitude (h in km) dependence of Rayleigh vertical extinction (magnitudes per unit air mass) is approximated by:
ARay (l,h) = 9.4977 x 10-3 x l -4 ((n- 1)l /(n-1)l =1) 2 x e -h/7.996 (1) where the scale height of the lower troposphere is taken to be 7.996 km and h = 2.369 km for the Roque de los
Muchachos Observatory.
The index-of-refraction term is given by: (n- 1)l/(n-1)l =1 = 0.23465 + (1.076 x 102)/(146 – l
-2) + 0.93161/(41 – l -2) (2)
2) Molecular Absorption
The major contributors are absorption bands of ozone and water vapour. The latter is not included in the calculated extinction curve, because the amount of water vapour above any observatory is extremely variable.
Water vapour bands can contribute more than 0.01 mag extinction per unit air mass at 7100, 8090, 9700 and 10800 A.
Vertical extinction by ozone is approximated by:
Aoz(l) = l.11 Toz Koz(l) (3) where Koz (cm-1) is the absorption coefficient (Gast 1960), and Toz (atm cm) is the total ozone above the observatory. Toz is independent of the observatory altitude, since atmospheric O3 is concentrated between 10 and 35 km. It does, however, exhibit seasonal variations and it can also vary significantly on time scales as short as a
few hours. The extinction curve given here uses a mean annual value of Toz appropriate to the latitude of La Palma, taken from Allen (1963).
The total vertical extinction coefficient A(l) for an aerosol-free atmosphere is then given by:
A(l) = ARay(l,h) + Aoz (l) (4) Values of A(l) have been calculated every 10 A between 3000 and 3500 A and every 50 A between 3500 and 11000 A, and are collected in Table 1. The extinction curve is shown in Figure 1, together with preliminary measurements obtained on dust-free nights (Andrews 1985, private communication).
Correction for Dust Extinction
To correct observations for total extinction, the contribution from aerosol scattering must be included. Available data (Jones 1984) suggest that to a first approximation the dust scattering at the Roque de los Muchachos observatory does not depend strongly on wavelength over the range considered here. In this case the dust correction term to the extinction curve can be deduced by comparing A(V), the theoretical extinction coefficient in the V band calculated as in (4) above, with ACAMC(V) the observed mean extinction coefficient in V measured on
the night of the observations by the Carlsberg Automatic Transit Circle group at the Roque de los Muchachos Observatory:
AAer = ACAMC(V) – A(V) (5) Values of ACAMC(V) for nights of interest can be obtained upon request from the Meridian Group at RGO Herstmonceux (in the first instance contact L V Morrison on ext. 3365). and will shortly be available on line from a file on the RGO Starlink VAX.
The total vertical extinction is then given by:
ATOT(l) = A(l) + AAer (6)
spacer
(PDF) Optical positions of radio stars observed with the meridian circle of the Real Instituto Y Observatorio de la Armada EN San Fernando
Positions of 53 radio stars observed with a meridian circle are presented. The positions of the radio stars are for the epoch of observation and the equinox J2000.0 and are in a system close to that of the FK5. The limiting magnitude is V = 9.5. The instrument is a classic visual meridian circle. The observation of transits of the stars is visual but automatically recorded; a photoelectric circle reading system stores the positions of the divided circle directly in computer memory. The mean error of a single observation in the zenith is 0.018 s in right ascension and 0.38 arcsec in declination. Comparisons are given of the catalogue with the FK5 and of radio stars positions with those of the Carlsberg Meridian Catalogue La Palma no. 4. Cross-references are given to other catalogues.
400 years of astrometry: from Tycho Brahe to Hipparcos …
Galileo Galilei’s use of the newly invented telescope for astronomical observation resulted immediately in epochal discoveries about the physical nature of celestial bodies, but the advantage for astrometry came much later. The quadrant and sextant were pre-telescopic instruments for measurement of large angles between stars, improved by Tycho Brahe in the years 1570–1590. Fitted with telescopic sights after 1660, such instruments were quite successful, especially in the hands of John Flamsteed. The meridian circle was a new type of astrometric instrument, already invented and used by Ole Rømer in about 1705, but it took a hundred years before it could fully take over. The centuries-long evolution of techniques is reviewed, including the use of photoelectric astrometry and space technology in the first astrometry satellite, Hipparcos, launched by ESA in 1989. Hipparcos made accurate measurement of large angles a million times more efficiently than could be done in about 1950 from the ground, and it will soon be followed by Gaia which is expected to be another one million times more efficient for optical astrometry.
![]() |
The BeginningsA General Overview of the Beginnings of the Roque de Los Muchachos ObservatoryRoque de Los Muchachos means BOYS ROCKS Smyth in 1856. Isaac Newton had written: “[Telescopes] cannot be so formed as to take away that confusion of rays which arises from the tremors of the atmosphere. The only remedy is a most serene and quiet air, such as may perhaps be found on the tops of the highest mountains above the grosser clouds.” Following this principle Smyth tested El Teide, the main peak of Tenerife, for its suitability to sustain astronomical observations. In the course of a voyage lasting 113 days in 1856, he spent 65 days at Guajara and Alta Vista on the slopes of El Teide at heights of 2,714m and 3,262m respectively. He performed tests of seeing by separating double stars and extended the magnitude limit of his 18cm refractor by 4 magnitudes to 14th magnitude from its habitual 10th magnitude at Edinburgh. He made meteorological observations of humidity, dew point, windspeed and dust-haze as well as numerous special astronomical observations designed to demonstrate the quality of the sky, for example of solar prominences, zodiacal light, and solar spectra. ![]() In January, 1967, Hermann Brück, Astronomer Royal for Scotland, first proposed the construction of a Northern Hemisphere Observatory (NHO) equipped with a 150-inch telescope. Exactly two years later, a committee under Fred Hoyle convened to examine the feasibility of such an undertaking, and by mid-1971 the project was in the planning stage. The choice of a site was strongly influenced by Merle Walker of Lick Observatory and his extensive investigation of the factors determining good observing conditions. Walker developed the technique of examining trails of circumpolar stars as a means of measuring the steadiness of the Earth’s atmosphere. He carried out such observations at sites around the world. Early in 1971 he wrote that “the best seeing occurs at sites on peaks near sea-coasts having cold ocean currents offshore that reduce the height of the [temperature] inversion layer, and where the laminar air-flow set up over the ocean still persists” (Walker, 1971). And he also concluded that “mountain peaks on (small) islands in warm oceans may be good sites, provided that the peaks are sufficiently high to place the observer above the inversion layer…” The importance of an inversion layer is that clouds get trapped below it, leaving clear skies above. On the basis of all the available evidence, Walker produced a list of potentially favourable observing sites that included Tenerife and its near neighbour La Palma, as well as Madeira, Corsica, and Crete. As is often the case in scientific endeavours, several people reached the same conclusion simultaneously. Walker was not alone in recognizing the Canary Islands’ potential. In 1971, John Alexander of the Royal Greenwich Observatory was investigating possible observing locations in the Mediterranean area, and his quest took him to La Palma in April 1971. His report, published later the same year, stated: “The ideal solution may be an international observatory site on the island of La Palma.” Moreover, European solar observers, joining in an association known as JOSO (Joint Organization for Solar Observations) visited Tenerife in 1971 March, and on their second visit in November 1971, flew in a light aircraft over the mountain top of La Palma testing for atmospheric turbulence with temperature sensors. The possible sites on La Palma were inspected by several JOSO site testers in summer and autumn 1971. The highest point at El Roque de Los Muchachos (2426 m) was selected as a possible site. The first observations on La Palma The first astronomical (solar) observations on the Roque de Los Muchachos peak were carried out by a JOSO team consisting of Göran Hosinsky, Lars Staveland and Hubertus Wöhl from 2 to 21 July 1972 (Hosinsky, Staveland and Wöhl, 1972). The British site-testing teams were dispatched to Tenerife and La Palma in 1972 under the direction of the Royal Observatory Edinburgh (ROE). The survey on Tenerife was made at the well-developed site of the Observatorio del Teide. Conditions on La Palma were very different. Rising to almost 8,000 feet at its highest point – called Roque de Los Muchachos – the caldera’s rim is a desolate region of scrub vegetation and volcanic ash. The site under investigation was Fuente Nueva, a small peak on the northern edge of the caldera adjacent to the Roque. The survey took place in August and September, 1972. This is an extract of probably one of the first publications with data taken at the Roque de los Muchachos Observatory (B McInnes, 1974) “The main work of the Project in the Canary Islands was done on the island of Tenerife; it seemed desirable to have some information also from the island of La Palma. A visit was made to the island during 1972 July 11 to 14 and decisions were made about the observational work that could easily be done there at that time. Dr Gough and Mr Heath then assembled the necessary equipment in Tenerife for a temporary outstation. They arrived at La Palma on July 28 with the Station 3 Land Rover carrying the equipment; construction work and transportation of the equipment to the site occupied the next few days; the observations began there on August 6 and ended on September 24. The highest point of the island is Roque de los Muchachos (2,423 metres, 7,949 feet). The site chosen for the observations was a peak called Fuente Nueva, situated about one kilometre north-east of Roque de Los Muchachos […] This site was selected by Dr Gough in the expectation that there might be a more favourable airflow there than at the higher Roque de Los Muchachos, since the prevailing wind is from the north and there is a fairly uniform slope with a gradient of about 1 in 4 from Fuente Nueva down to the coast, which is about 9 kilometres (5.5 miles) to the north. Observations of the seeing were made using a Lick Polaris Trail Telescope, which was mounted on a pier constructed of concrete blocks and local rocks cemented together. The objective of the Polaris Trail Telescope was about 4 metres (13 feet) above the general ground level. In general, seeing observations were made each hour during the night and meteorological observations were made at the beginning of each seeing observation. The air temperature was read from an accurate sheathed thermometer which was housed in a louvered box. The wind speed was measured with a hand-held anemometer, held at about the height of the telescope objective. The wind direction was obtained from a wind vane which was mounted at about 5 metres (16 feet) above the general ground level. Since there was no road to the site, access was on foot, with mules to carry equipment and supplies, and the observers were housed in small tents. The walk from the nearest vehicle road to the site took between two and three hours.” The results of this preliminary testing campaign were analyzed by Walker and Bennet McInnes of ROE and published in 1974. Their conclusion was that the “seeing conditions at Izaña, while good, were not excellent.” On the other hand, reports from Fuente Nueva “indicate conditions as good or better than those known at any other site.” Additional factors, such as the number of clear hours and the lack of light or atmospheric pollution singled out the La Palma site as being exceptional. The JOSO came to a similar conclusion. Professor Kiepenheuer of the Fraunhofer Institut in Freiburg, Germanyand JOSO leader became an enthusiastic advocate of La Palma at this time.
The mid 70’s Site Testing In 1974 August the Spanish authorities issued an invitation to form a Joint Astronomical Site Survey to undertake an international programme of site testing on the Canary Islands. A momentous meeting took place in 1974 December, when representatives of Denmark, Sweden, Germany and the United Kingdom were invited by the Rector of the University of La Laguna and the Presidents of the local Governments (Cabildos) of Tenerife and La Palma to discuss arrangements for the survey programme. Meanwhile, arrangements had already been made for the setting up of Polaris trail telescopes and meteorological equipment on La Palma and observations began on 25 November 1974 and ended in November1975 .
This encouraging progress came after a period of deep pessimism about the prospects for agreement on the use of La Palma. At the sixth meeting of the NHO Planning Committee in May 1973, Sir Martin Ryle complained about the lack of progress and suggested that UK universities could themselves move faster and more effectively both on the international and the technical questions. Dr M J Smith, who acted as a consultant to the Planning Committee, reported on Hawaii as almost the only possible site. Testing on Madeira (Ecumeada Alta peak) as an alternative started in October 1973. Spanish sites were no longer to be considered. Recovery from this deep gloom obviously depended on international politics, but it appears that several personal initiatives were also partially responsible. In March 1974 Prof S Edwards (Chairman of SRC) and Prof M F Walker visited the Consejo Superior de Investigaciones Científicas (CSIC) in Madrid and discussed the problem with Prof Masía, who was already well known for his help in international scientific affairs. Walker followed this visit with another in June. Prof F Sánchez, Director of the Observatory in Tenerife, had been encouraging the CSIC, the University and the local authorities to issue a joint invitation. A senior Spanish astronomer, Padre Romaña, was also active in overcoming the various political problems. At the meeting of December 1974, representatives of the various countries involved: Prof K O Kiepenheuer (Germany), Prof A A Wyller (Sweden), Dr K Gyldenkerne (Denmark), Prof H A Brück and F G Smith (UK) were shown the La Palma site from a light aircraft. F H Smith wrote: “A small hut with the waving figures of two site testers was the only sign of human activity on what is now a major European observatory. The weather was good and we were assured that this was typical. Everyone felt that this must be the site for the new observatory” (F G Smith, 1985). From that time onwards there was intensive action on three fronts: site testing, telescope design and the necessary international agreements. (see site tester’s notes, Rein Bakker, on their work on site). The meeting of 1974 December also revealed the intention of the Spanish astronomers and authorities to provide the basic facilities, ie a road, electrical power and water for the new observatory site. The project was to be regarded from the start as a cooperative venture, with the overseas participants providing telescope time and training for research astronomers in return for the use of the site and its facilities. The formal agreements necessary to secure the rights and specify the duties of the international participants were formulated two years later, when Prof Primo, President of the CSIC, invited representatives of research institutes in the various countries involved to Madrid. |
|||||||
![]() |
![]() |
||||||
Left: First work to build the first telescope in the Roque de Los Muchachos Observatory, the German Solar Tower in 1978 (photo by José Francisco Pérez Bravo). Right: German Solar Telescope in 1980 (photo by Lluís Tomas Roig). [ JPEG | TIFF ] |
![]() |
![]() |
Left: From left to right: Jacobus Kapteyn Telescope, Isaac Newton Telescope and the Polaris Trail Telescope used for seeing measurements during the site testing campaigns (picture taken in the early eighties). Right: The Polar Trail Telescope was erected on a pile of rocks and cement (construction on the left in the middle of the picture) [ TIFF ]. Another view: [ JPEG | TIFF ]. Both Pictures were taken by Nik Szymanek and Ian King in 1999. |
Planning the telescopes
The correct choice of instruments at an observatory is almost as important as its site. Observing time on large telescopes is notoriously oversubscribed. To devote all the available resources to a single large instrument would preclude observations that could be made with a smaller telescope. Thus, to make the best use of a good site requires the construction of a number of instruments of different apertures.
The idea of building a very large reflector for faint-object work was retained. However, difficulties are always encountered in constructing efficient auxiliary devices, such as spectrographs, if the primary mirror is too large. As a result, it was determined that an aperture of about 180 inches (4.5 metres) was the optimum size.
Upon investigating the availability of a suitable mirror, it was found that Owens-Illinois already had a 165-inch (4.2 metre) Cervit disk in stock. This was the last of a batch of blanks that included the primaries for the 3.9-metre Anglo-Australian Telescope and the Cerro Tololo 4-metre telescope. Each successive disk formed from the same mould came out slightly larger than its predecessor.
The option of using a different ceramic material, Zerodur, was discarded due to its high production cost (Goodsell, 1977), and slicing Owen’s blank to a 12 diameter-to-thickness ratio mirror, althoug feasible, was also discarded due to design constraints (Pope, 1978). A study of deflection and stress of this mirror was then carried out for the subsequent design of the supporting system (Mack, 1980).
Therefore economic and technical considerations led to the choice of the 4.2m blank for what was to become known as the William Herschel Telescope. In addition, the same reasoning suggested that an altazimuth mounting was appropiate for such a telescope. This decision reduced the weight of the mounting by 120 tons and halved the height of the dome needed.
While the WHT was being designed, new developments and ideas on telescope design came into the scene and as a consequence the design of the WHT was revised. After assesing all the possible options, it was decided to stay at the original design (Graham Smith, 1979) and purchase Owen Illinois’ blank.
In order to switch from primary focus to secondary mirro and avoid setting up a big crane to change the whole top end of the telescope, a novedous rotating top end ring was conceived (Pope, 1982).
Spectroscopy has been, and is certain to remain, one of the principal tools of astrophysical research. Very high resolution spectroscopy can be carried out efficiently with an instrument smaller in size than the Herschel. This type of work is well suited to a 100-inch telescope. Thus, it was decided to move the Isaac Newton Telescope from its cloudy Sussex home, while upgrading it with a new 100-inch Zerodur mirror, an improved mounting, and a large Coudé spectrograph (see the article “Castle in the Sky � The Story of the Royal Greenwich Observatory at Herstmonceux” by Chas Parker for more information on the already working Isaac Newton Telescope).
The third and smallest telescope at the NHO was to be a dual-purpose 1-metre reflector of novel design. The optics, devised by Charles Harmer and Charles Wynne of RGO, give the telescope a 1.5 degrees flat field for astrometric work. But, by removing a corrector and changing the secondary mirror, the system becomes a conventional Cassegrain for photometry or spectroscopy. Overall responsibility for the NHO project was put in the hands of the Royal Greenwich Observatory.
The proposals for these three telescopes were accepted by the Science Research Council in November 1974; planning and design started at RGO immediately. The project team at RGO was headed by Mr W. A. Goodsell, the chief telescope designer was Mr J. D. Pope, and the Project Scientist was Mr G. A. Harding.
Partnership: The Netherlands and Ireland
As the design of the telescopes and domes progressed, it became clear that the costs of building, maintaining and improving the ING would be too high for UK resources alone. So two options were considered, either reducing the scale of the UK installations or finding international partners who could help both with money and with manpower. Fortunately two such partners were found.
Dutch colleagues had already helped with site testing and they were aware of a forward-looking plan in which they hoped to build or gain access to new large telescopes overseas. The architect of the plan was Prof H van der Laan. At the IAU General Assembly in Montreal (1977) a basis for mutual advantage was worked out, and a momentous agreement was signed on 18June 1981 between the Netherlands Organisatie voor Zuiver-Wetenschappelijk Onderzoek (ZWO) and the UK SRC on cooperation in astronomy. Dutch colleagues became full partners, contributing both in cash and in manpower in proportion to the astronomical manpower in each country. The addition of 20% to the budget, with the addition of manpower to help commission the telescopes and to undertake the design and construction of instruments, allowed the project to continue undiminished. This partnership is symbolised by the naming of the 1-m telescope as the Jacobus Kapteyn Telescope. The collaboration is supervised by the Joint Steering Committee.
The Director of Dunsink Observatory, Prof P A Wayman, provided the introduction to the second partnership. It happened that the arrangements for Irish astronomers to use the Boyden Observatory in South Africa were terminating and it also happened that the Dunsink astronomers were particularly interested in the kind of astronomy that could be done with the new 1-m telescope. The result was an agreement between the Dublin Institute of Advanced Studies and the SRC, whereby Ireland would pay a proportion of the costs in return for observing time. Technical help would also be provided by Dunsink; this agreement was immediately put into action with great benefit to the UK instrument designers.
La Palma is chosen
Analysis of all the testing data revealed clearly that Mauna Kea and Fuente Nueva were the best astronomical sites known. The difference between the two was slight. For example, the seeing was better than 1 arc second 36 percent of the time on Mauna Kea and 40 percent for La Palma. The two really significant drawbacks of the Hawaiian site for the British were the problems that observers would suffer from its much greater altitude and its distance from Europe. So, after many years searching, La Palma was finally chosen.
On 26 May, 1979, almost 10 years after the first committee met to consider a Northern Hemisphere Observatory, a treaty governing its establishment was signed (The “Acuerdo Internacional en Materia de Astrofísica”, BOE, 161, 6 July 1979). Spain agreed to let Denmark, Sweden, and Britain build on La Palma, in exchange for 20 percent of the observing time.
On the Spanish side, a major reorganization of astronomical research had led to the founding of the Instituto de Astrofísica de Canarias (IAC) under the directorship of Francisco Sánchez. The IAC owns and operates on behalf of Spain the Izaña observatory and the new site on La Palma – now officially known as the Roque de Los Muchachos Observatory.
The Danish contribution is the Carlsberg Automatic Transit Circle. The Swedes wanted to build a 60-cm stellar telescope and a solar tower with a 60-cm heliostat and 44-cm Cassegrain. In fact, construction of these installations started within days of the treaty’s signing.
Photos of the construction and erection of the ING telescopes can be found here:
Pollution of the night sky
It’s interesting to note that since the beginning astronomers were concerned about conserving the good Palmeran sky conditions. The following is a good example of the latter (Gietzen, 1982): “One of the reasons for selecting La Palma as the site for the installation of the UK’s major optical telescopes was the comparitively minor interference with the quality of the night sky by urban lighting and overflying aircraft. Any increase in either of these factors will lead almost inevitably to a reduction in the observational utility of the site[…].
I would like co-operation in identifying ‘early warnings’ in order that action may in some instaces be started sooner.
There are two areas where every one can contribute:
1) I would like to be informed of any reports, whether in newspapers or elsewhere, of possible future increases in the level of artificial lighting in the island.
2) I would also like to start a record of the incidence of aircraft vapour trails in the vicinity of the site. Reports to be included in this would include date and time of observation and position and direction in at least crude terms. Regular visitors to the site are requested to co-operate in this.”
In 1988 the Spanish Government approved the Sky Law in order to protect the Palmeran sky. The Oficina para la Protección del Cielo was then set up.
Name of the British telescopes on La Palma
At the meeting of the International Scientific Committee on Tenerife on 25 May 1984, Alec Boksenberg announced that the 1 metre telescope had been named the Kapteyn Telescope after Jacobus Kapteyn, the Dutch astronomer. The name was appropiate since he had worked on problems of galactic structure similar to those which the telescope was expected to attack. He also announced that the interim name UK Optical Telescopes woud be replaced by the name Isaac Newton Group. In the 1960’s Britain’s biggest telescope was named after the British scientist most highly regarded in the world. In the 1980’s its best collection of telescopes continued to commemorate this man (Murdin, 1984).
The royal inauguration
The observatory on La Palma was inaugurated by seven heads of state, or their representatives, on 29 June 1985. Attending were King Juan Carlos of Spain, Queen Beatrix of the Netherlands, Queen Margrethe of Denmark, King Gustav of Sweden, the Presidents of West Germany and of Ireland, and the Duke of Gloucester representing Queen Elizabeth of the United Kingdom. After ceremonies and banquets on Tenerife on June 28, the royal party and the guests flew to La Palma on the morning of the 29th, to ascend to the Roque de los Muchachos in perfect weather. The heads of state travelled from telescope to telescope, dedicating each in front of a small audience. The main ceremony, inaugurating the whole observatory, took place in a large open-air auditorium. The audience included royal guests, astronomers, engineers, Nobel Laureates, national and local dignitaries, Spanish, Dutch, Danish, Swedish and British staff on the Roque, civil servants, military officials and media representatives, in a gathering in which protocol played a relatively minor part. Other events on the island included the opening of the Convent of San Francisco in which there was displayed an exhibition of Hispano-Arabic astronomical instruments and maps, some loaned by the Old Royal Observatory, Greenwich.
![]() |
![]() |
spacer
spacer
New quantum teleportation record paves the way towards a worldwide quantum network



