As you view this post you may find yourself in a state of disbelief. Trust me, I understand. When I first learned about this stuff and a lot other things that have been revealed to me, I had the same reaction. I beg you, don’t let your discomfort with the material cause you to reject it out of hand. Stay with me. This stuff is REAL!! More real than the world you see around you everyday.
If you are new to my webpage, go back through all the past posts and take your time to view them all. It helps to walk through the information step by step. I have been blessed to live through these developments as they came to light. I have been serving the Lord for over 50 years. I have been documenting these truths and sharing them online for over 20 years.
When I first learned about CRISPR I was amazed at how it clearly was the source of a lot of what we were seeing in our World. I created the CRISPR series below. This post is a continuation of that series.
CRISPR – Part 1- GENETIC MANIPULATION the Springboard
CRISPR – Part 2- The DESTROYER
CRISPR – Part 3- THE ULTIMATE END
CRISPR – Part 4 – Unleashed
CRISPR-WUHAN VIRUS-WAKE UP and READ THE SIGNS!
CRISPR – Part 5 – OUR FUTURE
spacer

Source: Nephtali1981

Note: Look at this guys great research and description incl links below… CRISPR!
Timestamps:
00:00 Introduction00:55 Genetics02:43 Genetic Engineering05:01 Cloning09:53 Chimera37:36 Superheroes
Genetics is the study of genes, genetic variation, and heredity in organisms.
It is an important branch in biology because heredity is vital to organisms’ evolution.
Gregor Mendel, a Moravian Augustinian friar working in the 19th century in Brno, was the first to study genetics scientifically.
Mendel studied “trait inheritance”, patterns in the way traits are handed down from parents to offspring over time.
He observed that organisms (pea plants) inherit traits by way of discrete “units of inheritance”.
This term, still used today, is a somewhat ambiguous definition of what is referred to as a gene.
Genetic engineering, also called genetic modification or genetic manipulation, is the modification and manipulation of an organism’s genes using technology. It is a set of technologies used to change the genetic makeup of cells, including the transfer of genes within and across species boundaries to produce improved or novel organisms.
CRISPR is a family of DNA sequences found in the genomes of prokaryotic organisms such as bacteria and archaea. These sequences are derived from DNA fragments of bacteriophages that had previously infected the prokaryote. They are used to detect and destroy DNA from similar bacteriophages during subsequent infections. Hence these sequences play a key role in the antiviral (i.e. anti-phage) defence system of prokaryotes and provide a form of acquired immunity. CRISPR is found in approximately 50% of sequenced bacterial genomes and nearly 90% of sequenced archaea.
According to Greek mythology, the Chimera, Chimaera, or Chimæra was a monstrous fire-breathing hybrid creature from Lycia, Asia Minor, composed of different animal parts. It is usually depicted as a lion, with the head of a goat protruding from its back, occasionally depicted with dragon’s wings, and a tail that might end with a snake’s head.[3] It was an offspring of Typhon and Echidna and a sibling of monsters like Cerberus and the Lernaean Hydra.
The Nephilim are mysterious beings or people in the Hebrew Bible who are described as being large and strong. The Hebrew word Nephilim is sometimes translated as “giants”, and sometimes as its literal meaning “the fallen ones”. Their origins are disputed. Some, including the author of the Book of Enoch, view them as offspring of fallen angels and humans. Others view them as offspring of the descendants of Seth and Cain.
spacer
Safely Inhibiting Gene Editors
DARPA’s Broad-Spectrum Antagonists For Editors, or B-SAFE, program aims to identify and optimize novel molecules that enable precise, tunable control of gene editing processes

One promising avenue to enhance safety, efficacy, and utility is the discovery or design of novel inhibitors which have the potential to obstruct and tune regulation of CRISPR-mediated and other genome editing tools by limiting unintended, off-target effects and enabling control of activity in time and space. The DARPA Broad-Spectrum Antagonists For Editors, or B-SAFE, (oh for sure, because we KNOW just how DARPA can be trusted! Certainly now we are set at ease knowing DARPA is handling it. NOT!!) program aims to develop platform technologies for highly potent inhibitors for multiple classes, types, and species of editors with enhanced activity, utility, and breadth of coverage. By harnessing advanced computational discovery capabilities such as deep learning, the program also seeks to develop a platform for rapid discovery and development of inhibitors of novel, emergent gene editor technologies.
“Previous DARPA investments in the Safe Genes program demonstrated discovery of potent inhibitors for a wide array1 of CRISPR-Cas technologies, and developed platforms for discovery of inhibitors of these systems2,3,” said Dr. Shannon Greene, B-SAFE program manager. “The B-SAFE program seeks to leverage these and other efforts to develop tools for discovery, optimization, and validation of broad-spectrum inhibitors for gene editing technologies.”
If the 30-month B-SAFE program is successful, performers will demonstrate broad-spectrum, potent and durable solutions as outlined in the broad agency announcement. Speed – both from novel editor to candidate inhibitor discovery, and from novel editor to in vitro model – is also critical, as is inhibitor functionality for novel editors.
DARPA will assess novel inhibitor activity in vitro over the course of the program and a subset of top performing molecules will be selected for scale up at quantities sufficient for testing and evaluation by Department of Defense stakeholders. Performers will also engage with appropriate regulatory authorities to meet or exceed safety regulations. Teams may be required to meet with ethical, legal, and societal implications experts and ensure the research addresses any related concerns.
“In concert, DARPA is interested in exploring methods to rapidly discover inhibitor molecules for novel gene editing technologies beyond CRISPR-Cas systems to keep pace with the rapidly advancing field while promoting safe use and minimizing off-target effects.” added Greene.
A special notice is now available on SAM.gov.
[1] Marino et al., Anti-CRISPR protein applications: natural brakes for CRISPR-Cas technologies. Nat. Methods 2020, 17(5), 471-479.
[2] Maji et al., A High-Throughput Platform To Identify Small-Molecule Inhibitors Of CRISPR-Cas9. Cell. 2019, 177, 1067-1079.
[3] Lim, et al., A general approach to identify cell-permeable and synthetic anti-CRISPR small molecules. Nat. Cell Biol. 2022, 24, 1766-1775.
spacer
Genetically Engineering the Nervous System with CRISPR-Cas
Abstract
The multitude of neuronal subtypes and extensive interconnectivity of the mammalian brain presents a substantial challenge to those seeking to decipher its functions. While the molecular mechanisms of several neuronal functions remain poorly characterized, advances in next-generation sequencing (NGS) and gene-editing technology have begun to close this gap. The clustered regularly interspaced short palindromic repeats (CRISPR)-associated protein (CRISPR-Cas) system has emerged as a powerful genetic tool capable of manipulating the genome of essentially any organism and cell type. This technology has advanced our understanding of complex neurologic diseases by enabling the rapid generation of novel, disease-relevant in vitro and transgenic animal models. In this review, we discuss recent developments in the rapidly accelerating field of CRISPR-mediated genome engineering. We begin with an overview of the canonical function of the CRISPR platform, followed by a functional review of its many adaptations, with an emphasis on its applications for genetic interrogation of the normal and diseased nervous system. Additionally, we discuss limitations of the CRISPR editing system and suggest how future modifications to existing platforms may advance our understanding of the brain.
Introduction
Complex behavior is driven by extensive structural and genetic interactions in the mammalian CNS. Historically, neuroscientists have examined these interactions using a variety of histological, electrophysiological and pharmacological techniques. While indispensable, these techniques lack the specificity of targeted genetic approaches to dissect neuronal function. Recent advances have allowed the coupling of high-throughput next-generation sequencing (NGS) technologies with the cell-type specificity of modern molecular genetics to interrogate complex network interactions and behaviors at unprecedented scale and resolution. The ability to read, write, and manipulate genomes with cell-type specificity is critical, especially considering the cellular heterogeneity of various CNS structures (Chung et al., 2005).
Early attempts at targeted gene editing were performed with zinc finger nucleases (ZFNs) and transcription activator-like effector nucleases (TALENs), both of which rely on programmable DNA-binding proteins coupled to active endonucleases to cleave specific DNA sequences (Kim et al., 1996; Carroll, 2011; Joung and Sander, 2013). While suitable for a variety of applications (Gaj et al., 2013), these systems have fallen out of favor for new genome editing systems due to relative disadvantages such as their extensive protein engineering requirements. Recent advances in gene editing technology have culminated in the discovery of clustered regularly interspaced palindromic repeats (CRISPR)-Cas9, a bacterial immune system which has been repurposed for mammalian genome editing applications (Jinek et al., 2012). Unlike its predecessors, CRISPR nucleases target DNA in an RNA-directed manner, using a programmable single guide RNA (sgRNA) to target complementary DNA sequences for cleavage.
Since the initial adaptation of CRISPR, novel variants continue to be discovered in diverse microbial species, differing in endonuclease size, substrate preference and target recognition requirements (Ran et al., 2015; Abudayyeh et al., 2017). Moreover, several nuclease variants have been engineered for expanded targeting capacity and improved fidelity (Kleinstiver et al., 2015, 2016; Slaymaker et al., 2016; Chen et al., 2017). Perhaps most versatile are the catalytically inactive variants designed to function as DNA-binding proteins, which can regulate transcription, modify the epigenome, target RNA for destruction and facilitate base-editing through the action of their coupled enzymatic domains (Dominguez et al., 2016; Rees and Liu, 2018; Pickar-Oliver and Gersbach, 2019). The highly flexible and multifunctional character of this platform has established CRISPR-Cas as the predominant genome editing system in use today. Here, we provide an overview of CRISPR-Cas technology, followed by a review of its many adaptations for genetic interrogation and modification. Throughout this article, we emphasize applications of CRISPR systems in the field of neuroscience and discuss the potential of this technology to advance our understanding of the brain.
CRISPR-Cas
Isolated from Streptococcus pyogenes, the Type II CRISPR-Cas9 system (spCas9) was the first enzyme repurposed from its native role as a bacterial adaptive immune system for genome editing applications in eukaryotic cells (Jinek et al., 2012). While spCas9 remains the most popular CRISPR nuclease, various CRISPR-Cas systems with divergent structures and properties have been discovered. These systems are broadly categorized by their nuclease composition, with those containing multisubunit nuclease structures pertaining to Class 1 and those composed of a single protein pertaining to Class 2. Within Class 2, systems are further subdivided into Types II, V, and VI, which pertain to DNA-targeting Cas9 and Cas12a and RNA-targeting Cas13, respectively (Shmakov et al., 2017). As Class 2 systems have been used in the majority of neuronal gene editing experiments, they will therefore be the focus of this review. Class 1 systems and their uses are described elsewhere (Cameron et al., 2019; Pickar-Oliver et al., 2019).
The prototypical CRISPR nuclease, spCas9, is an RNA-guided DNA endonuclease that relies on an RNA duplex comprised of a CRISPR RNA (crRNA) and a transactivating crRNA (tracrRNA) for its activity (Fig. 1A). CRISPR RNAs direct Cas9 enzymes to their intended genomic targets, whereas tracrRNAs are responsible for stimulating Cas9’s endonuclease activity and mediating pre-crRNA processing and maturation. Although discovered as two distinct RNAs in nature, it was experimentally determined that the essential elements of the tracrRNA-crRNA duplex could be combined into a chimeric sgRNA. Therefore, genome editing using this system only requires the Cas9 protein and the sgRNA. Cas9-DNA targeting occurs when the Cas9-bound sgRNA hybridizes to its target-DNA proximal to a short sequence known as the protospacer adjacent motif (PAM), which is used for target recognition. Once Cas9 binds to the genomic target site, it creates a double stranded break (DSB) approximately three bases upstream of a PAM-containing locus with sufficient crRNA complementarity. DSB formation initiates the nonhomologous end joining (NHEJ) DNA repair mechanism, which, due to the error prone nature of this repair pathway, creates insertion and deletion (indels) mutations at the DSB site (Fig. 1A). If the DSB occurs within the protein coding region of a gene, a loss of protein function can occur. The deletion of relevant codons or a shift in the reading frame often creates a truncated protein, collectively leading to a null allele/gene knock-out (KO; Jinek et al., 2012; Cong et al., 2013; Mali et al., 2013). Alternatively, if a donor DNA template is provided, homology-directed repair (HDR) can occur instead of NHEJ. This phenomenon can be harnessed to specifically modify the genome at precise loci (Fig. 1B; Cong et al., 2013; Mali et al., 2013; Wang et al., 2013). However, HDR-mediated DNA repair via existing technology remains very inefficient, and therefore, its use in non-dividing cells (i.e., neurons) in vivo has limited utility (Chu et al., 2015; Maruyama et al., 2015).
CRISPR-Cas9 mediated genome editing. A, Cas9 target recognition occurs through sequence complementarity between a Cas9-associated sgRNA and a genomic target sequence. Target recognition requires the presence of a proximal 3′ PAM, which facilitates Cas9 binding and endonucleolytic cleavage. Cas9’s dual catalytic domains, HNH and RuvC1, mediate complementary and non-complementary strand cleavage, respectively. DSBs repaired by NHEJ can introduce short insertion/deletion (indel) mutations that cause frameshifts capable of disrupting protein coding sequences, causing loss of gene function. Alternatively, HDR can be used for site-specific, sequence alteration by supplying DNA templates encoding user-specified modifications. B, The vSLENDR and HMEJ knock-in strategies exploit homology-dependent repair pathways to introduce foreign sequences. vSLENDR and HMEJ both require long homology arms flanking the DSB site for efficient gene insertion. However, HMEJ utilizes a hybrid NHEJ/HDR strategy which departs from the HDR-based vSLENDR strategy by also requiring DSBs to release the donor DNA template (2B – Red arrows). C, Homology-independent (NHEJ) knock-in strategies mediate sequence insertion by forming DSBs at desired target sites and donor templates simultaneously. HITI utilizes a donor template that is flanked by sgRNA recognition sites that match the genomic target. Simultaneous donor/target cleavage and repair stimulate donor template insertion. HiUGE also requires simultaneous donor and target cleavage; however, HiUGE donor vectors encode both a donor template and a self-targeting sgRNA.
The Type V nuclease Cas12a (also known as Cpf1, CRISPR from Prevotella and Franciscella 1), is a related DNA targeting enzyme that departs mechanistically from Cas9 in ways that may be advantageous. For example, unlike Cas9, Cas12a processes its own CRISPR array (crRNA precursors) into mature crRNAs, independent of any ancillary enzymes and a tracrRNA. Cas12a also recognizes a different PAM sequence (Cas12a, TTTN; Cas9, NGG), generates staggered cuts and requires a much shorter guide RNA than Cas9 (∼40 nt, Cas12a; ∼100 nt, Cas9). Cas12a’s compact guide RNA architecture and self-crRNA processing ability make it well suited for multiplexed gene-targeting, particularly through the use of custom arrays encoding multiple crRNAs. Recently, these properties were optimized and harnessed for large scale gene-editing, with Campa and coworkers reporting the ability to deliver and express 20 crRNAs and Cas12a from a single vector, simultaneously (Campa et al., 2019). The continued discovery and development of new CRISPR-Cas systems with advantageous properties is highly encouraging for the future of biomedical research and therapeutic development.
Gene Disruption in the Mammalian Brain via CRISPR-Cas and NHEJ
Targeted gene disruption is a popular approach for dissecting the functional role of many synaptic and neuronal proteins in vivo (Gray et al., 2011; Uezu et al., 2016). Historically, this has required conventional mutant germline engineering, which is experimentally time-consuming, can generate deleterious phenotypes, and is generally prohibitive for multigene perturbation. Gene disruption with CRISPR-Cas has been demonstrated as a promising alternative to existing gene KO strategies. Several groups have begun to apply CRISPR-Cas to disrupt genes in mature neurons in vitro and in vivo, by targeting Cas9 to specific loci and relying on NHEJ repair pathways to create indels, leading to a high rate of gene disruption (Incontro et al., 2014; Swiech et al., 2015).
The earliest studies that implemented CRISPR-Cas for neuronal gene editing in vivo established the lack of toxicity of prolonged Cas9 expression in neurons while also creating the first transgenic and viral platforms for their expression and delivery (Platt et al., 2014; Swiech et al., 2015). Using these transgenic mice, Platt and coworkers also demonstrated the high KO frequencies (84% biallelic, 9% monoallelic; NeuN) achievable in neurons transduced with AAV-sgRNAs. Swiech and coworkers sought to expand the applicability of CRISPR for broad in vivo use by adapting Cas9 for packaging into popular viral vectors for gene delivery into the brain (Swiech et al., 2015). The adeno-associated virus (AAV) DNA packaging limit (∼5 kb) is a major limitation for viral delivery in vivo, therefore packaging the Cas9 transgene (∼4 kb), sgRNA cassette and other necessary expression components into a single vector is infeasible. To circumvent this, Swiech and coworkers developed an AAV-CRISPR system that expresses spCas9 and its respective sgRNA from separate AAV vectors. Applying AAV-CRISPR to target various genes in vitro and in vivo recapitulated the substantial editing efficiency observed in transgenic Cas9 mice. For example, targeting methyl CpG binding protein 2 (MeCP2) in cultured neurons produced morphologic defects concurrent with MeCP2 loss of function. Furthermore, multiplexed targeting of several DNA methyltransferase genes within the dentate gyrus was capable of producing context-specific freezing deficits in mice that received contextual fear conditioning, while sparing behavioral performance in other tasks (open field test, novel object recognition, elevated plus maze).
Traditional gene editing strategies have relied heavily on engineered viral vectors for in vivo construct delivery (Yin et al., 2017). Although AAV and lentiviral (LV) vectors are widely used for their ability to stably express transgenes for extended periods, the potential drawbacks of viral delivery and prolonged Cas9 expression for therapeutic gene editing have received increased attention. For example, higher cellular concentrations of Cas9 have been shown to decrease specificity, presumably because off-target cleavage is the only possibility after all target sites have been destroyed (Davis et al., 2015). This observation has raised concerns for therapeutic developments that rely on viral gene transfer, which in the case of AAV-mediated gene expression, persists for several years after delivery (Nathwani et al., 2011; Wojno et al., 2013; Colella et al., 2018; Guilbaud et al., 2019). Engineered ribonucleoprotein complexes (RNP; Cas9 protein bound to a guide RNA) and Cas9-encapsulating nanoparticles have been developed as non-viral alternatives for local, transient CRISPR expression in the brain.
Staahl and coworkers introduced a cell permeable Cas9-RNP capable of transient and titratable gene disruption (Staahl et al., 2017). Cas9-RNPs were designed with repeating SV40 nuclear localization sequences (NLS), which have been previously reported to enhance cell-penetrance (Liu et al., 2015). Preassembled Cas9-RNPs were injected into the S1 primary somatosensory cortex, the V1 primary visual cortex, the dorsal striatum and the hippocampus of Ai9-tdTomato mice. Reporter activation increased in a dose-dependent manner with larger administered doses of Cas9-RNP. Furthermore, RNP injection into the dorsal striatum did not induce a significant immune response, which has been a point of concern after reports of anti-Cas9 immune responses (Chew et al., 2016).
Recently, the nanoparticle-based CRISPR-Gold system was used to target mGluR5, a metabotropic NMDA receptor involved in autism spectrum disorder (ASD)-related hyperexcitation (Lee et al., 2018). CRISPR-Gold RNPs containing mGluR5-targeting guides were infused into the striatum of fragile X mental retardation 1 (FMR1) KO mice, which significantly reduced exaggerated stereotypies (excessive digging and jumping). Analysis revealed 14.6% of striatal mGluR5 genes contained loss of function (LOF) mutations, while mGluR5 mRNA and protein levels decreased by roughly 50%. Despite modest editing efficiency, these results highlight the potential of nanoparticle-based systems to deliver CRISPR and therapeutically edit genes in the brain. While CRISPR-Gold administration was sufficient to reverse the behavioral phenotype, additional optimization of nanoparticle entry into neurons will likely expand the use of non-viral, nanoparticle-based methods for genome editing in neuroscience.
Another group engineered membrane-permeable nanocomplexes to deliver Cas9 RNPs into the brain (Park et al., 2019). CRISPR nanocomplexes were generated by fusing an amphiphilic R7L10 peptide to Cas9 RNPs to permit cellular entry. R7L10-Cas9-RNPs exhibited remarkable in vivo stability and longevity, sustaining high levels of expression for over a week, which declined below detection thresholds after three weeks. Unlike virally delivered CRISPR transgenes that remain stably expressed for extended periods, nanocomplex-delivered RNPs possess limited opportunity to perform their gene targeting functions. Remarkably, in vivo targeting of β-secretase 1 (Bace1) in the hippocampal CA3 region of 5XFAD transgenic mice produced an editing efficiency of 45% which significantly reduced Aβ plaques and Aβ42 secretion. Surprisingly, a single hippocampal injection of Bace1-targeting nanocomplexes elicited persistent improvements in contextual and associative memory three months after treatment (Park et al., 2019). While the decay rates of injected RNPs and their potential off-targeting effects remain to be determined, additional research could accelerate the development of injectable RNP therapies for focal neurologic disease.
Germline Editing with CRISPR
Genetically modified animals have been instrumental in understanding genetic contributions to neuronal development, function and disease. Conventionally, establishing transgenic animal strains has been a time- and labor-intensive process that requires several months and specialized facilities for completion (Capecchi, 2005). In recent years, many of these constraints have been overcome by CRISPR-Cas9 genome editing. The ability to rapidly produce transgenic animals harboring multiple germline mutations with relative ease is a significant improvement over traditional transgenic animal production approaches. For a more detailed discussion on generating transgenic/knock-in mice with CRISPR-Cas, we direct the reader to the following articles (Yang et al., 2014; Henao-Mejia et al., 2016; Williams et al., 2016).
While the broad availability of genetically modified mice has contributed to their widespread use in biomedical science, rats remain the preferred animal model in behavioral neuroscience research. The paucity of available transgenic rat models has left an unmet demand for additional transgenic rat lines (Ellenbroek and Youn, 2016). Germline genome editing with CRISPR-Cas9 has emerged as a highly efficient method for producing transgenic strains. As such, CRISPR-Cas9 was used to generate transgenic Cre-dependent Cas9 and Cre-dependent Cas9-nickase [Cas9(D10A)] rats and an improved Cre recombinase (iCre) rat line regulated by the dopamine transporter promoter (DAT-iCre) (Bäck et al., 2019). To show that gene targeting was Cre dependent, Back and coworkers infused AAVs encoding iCre and tyrosine hydroxylase (TH)-targeting sgRNAs into the midbrain. Four weeks after infusion, a 45% and 60% decrease in TH immunoreactivity was observed in the substantia nigra and striatum, respectively. To determine the targeting efficiency achievable with double-transgenic animals (DAT-iCre+/Cas9+), AAVs encoding Manf sgRNAs were infused into the midbrain. After four weeks, only 3% of dopaminergic neurons demonstrated Manf immunoreactivity; additionally, nearly 90% of non-dopaminergic neurons remained Manf+, thereby illustrating the potential of these lines to facilitate highly specific genome editing with extremely high editing efficiencies. With the availability of neuron-specific Cre-driver lines (GABAergic, D1, D2, parvalbumin), these Cre-dependent Cas9 rat lines present a significant advancement for future gene studies in behavioral neuroscience.
Gene Modification in the Mammalian Brain via CRISPR-Cas and HDR
Currently the factors governing DNA repair pathway choice remain unclear. In general, NHEJ appears to be far more efficient and active compared to HDR (Cox et al., 2015). It is generally believed that HDR is mostly restricted to the S/G2 phases of the cell cycle, which may restrict harnessing HDR’s full potential in postmitotic cells such as neurons (Saleh-Gohari and Helleday, 2004). This may be due to phase specific conditions favorable to recombination such as the presence of proximal sister chromatids or the increased expression of requisite repair machinery. Both are conditions which may preclude robust HDR activity in terminally differentiated neurons. However, this remains to be determined.
Although Cas9’s canonical function is to cleave DNA, it can also be used to introduce foreign transgenes and new sequences using HDR (Fig. 1B). Low neuronal HDR activity has largely discouraged gene-editing attempts in the brain. However, recent evidence has surfaced demonstrating the successful modification of neuronal genes in vivo. Using their newly developed CRISPR-based HDR system dubbed SLENDR (single-cell labeling of endogenous proteins via HDR system), Mikuni and coworkers targeted neural progenitors at embryonic days (E)12 and E15, when HDR should be active. Embryonic brains were subjected to in utero electroporation (IUE), to deliver sgRNAs, Cas9 coding plasmids, and a hyperactive Piggyback transposase system to allow the stable integration of these transgenes and donor templates consisting of single stranded oligonucleotides (ssODNs). This approach enabled the modification of targeted genes to possess N-terminal or C-terminal epitope tags. Remarkably, SLENDR was also capable of inserting large sequences into endogenous loci, such as the GFP-coding region, facilitating protein localization studies. The authors reported modification frequencies as high as 7.5% of neurons when targeting was performed at E12, and slightly lower levels when performed at E15. It is important to note that indel formation will occur at a much higher frequency compared to HDR-mediated sequence insertion using this system. Additionally, the authors specifically targeted the beginning and end of the protein coding regions, to reduce the chance that indel formation would have a consequence on protein structure and function.
Nishiyama and coworkers adapted the SLENDR system for viral delivery. This system, referred to as vSLENDR (AAV/CRISPR-based viral-mediated single-cell labeling of endogenous proteins via HDR system), was shown to allow HDR-mediated gene modification of neurons in the mouse adult brain (Fig. 1B). They observed gene modification efficiencies in vivo (Nishiyama et al., 2017) as high as ∼30% of targeted neurons, which provides proof-of-principle for HDR-mediated editing in mature neurons. While encouraging, the mechanism of HDR-mediated editing requires additional characterization and subsequent optimization before it can be broadly applied for in vivo studies.
Additional Gene Modification Strategies
While broadly considered an inherently error-prone process, various NHEJ-dependent DNA-editing tools have been developed that demonstrate the remarkably high editing frequency and precision of NHEJ repair (Fig. 1C). These tools, designated homology-independent (HI) targeted insertion (HITI), Homology-Mediated End Joining (HMEJ) and Homology-independent Universal Genome Engineering (HiUGE) have been shown to effectively integrate exogenous DNA sequences at similar frequencies (20% to over 50%). The first of these, HMEJ, exploits homology-dependent (HD) processes by coupling donor templates harboring sgRNA recognition sites with targeted, Cas9-mediated DNA cleavage. HMEJ-DNA donors contain 5′ and 3′ distal sgRNA sites that, upon cleavage, release a long donor cassette which encourages integration into the cleaved genomic site. When applied to adult mouse neurons in vivo HMEJ produced knock-in frequencies of ∼50%. Although HD strategies ensure locus specificity through extensive donor template homology, unique template production is generally restrictive for high-throughput experimentation. Therefore, unrestricted by locus homology, HI systems have gained more traction. The HITI and more recently developed HiUGE systems also exploit NHEJ repair to introduce DNA payloads. Both HITI and HiUGE incorporate similar components and mechanisms to achieve targeted transgene integration. For example, the use of a non-homologous donor vector with sgRNA recognition sequences is ubiquitous among NHEJ-mediated systems. However, HITI and HiUGE depart as HiUGE donors contain self-targeting sgRNAs, while HITI donors require sgRNA recognition sequences to be manually matched between the target and donor. The addition of a self-targeting guide RNA to HiUGE vectors permits the development of “all-in-one” donor libraries that may function complimentarily with large-scale CRISPR genetic screens.
Regulable Gene Editing with Inducible CRISPR-Cas Systems
Germline editing with CRISPR-Cas9 has proven remarkably useful for genetically modifying animals (Li et al., 2013; Chapman et al., 2015; Remy et al., 2017). However, germline modifications can produce undesirable developmental phenotypes providing little benefit for studies interrogating gene function in adult animals. Furthermore, temporally precise manipulations may be required for studying gene function in dynamically regulated processes. In such situations it may be beneficial to deploy temporally regulable systems capable of gene editing within tightly restricted windows. Towards this aim, CRISPR-Cas9 has been combined with several other technologies to develop systems that can be regulated genetically, optically, or with small molecules (Dow et al., 2015; Zetsche et al., 2015).
Some of the first inducible CRISPR systems were regulated by components of the popular tetracycline-dependent promoter (Tet) system (Dow et al., 2015; de Solis et al., 2016), which can be regulated in Tet-on (rtTA) and Tet-off (tTA) configurations (Fig. 2; Gossen and Bujard, 1992; Gossen et al., 1995). de Solis and coworkers developed the first doxycycline (Dox)-inducible Cas9-based editing system that saw use in the brain. First, Cas9 was placed under the control of the Dox-dependent TRE3G promoter in an to attempt to temporally regulate Cas9 expression and subsequent genome editing (de Solis et al., 2016). However, TRE3G-driven Cas9 exhibited leaky expression in vitro, prompting the development of regulable sgRNA expression vectors, which successfully regulated gene-editing events in a Dox-dependent manner. To determine whether this Dox-regulable CRISPR-Cas9 system was suitable for in vivo applications, AAV vectors encoding Cas9 and Dox-inducible sgRNAs were infused into the basolateral amygdala (BLA). In vivo genome-editing analysis revealed that only animals receiving Dox contained indels at the target locus. Additionally, Dox-inducible and constitutively expressed systems exhibited near identical levels of gene editing, demonstrating that spatiotemporally precise editing is achievable in the brain without significant loss of efficiency. Additional Cre and Dox-inducible CRISPR systems have been developed based on the smaller SaCas9 endonuclease. For further discussion of the SaCas9 orthologue and these inducible tools, we direct the reader to (Kumar et al., 2018; Zhou et al., 2018b).
Inducible CRISPR-Cas systems. CRISPR-Cas9 genome editing can be spatially and temporally regulated with a variety of genetic, small molecule, and optical techniques. Intein-Cas9 and iCas can be regulated with the small molecule 4-hydroxytamoxinfen (4-HT). Whereas 4-HT-induced intein splicing renders Intein-Cas9 constitutively active, iCas is bidirectionally regulable. Gene targeting sgRNAs can be transcriptionally regulated with the Dox response H1/TO promoter. Additionally, both sgRNA and Cas9 expression cassettes can be rendered Cre dependent with the insertion of flanking loxP sites. Split architecture Cas9 systems have also been rendered photoinducible through fusions to light responsive, heterodimerizing molecules.
While the conditional Tet- and Cre- based systems are frequently used to restrict gene expression temporally and spatially, their specificity and regulation is largely transcriptionally mediated. In cases where swift gene-editing is desirable, it is beneficial to reduce the response rate of the system. Post-translationally regulated processes circumvent the de novo transcription and translation involved in transcriptionally mediated responses, permitting a more rapid response to dynamic cellular environments. Additionally, reducing the permissible window for gene-targeting events could significantly reduce the off-target modifications reported with constitutively active Cas9. Several inducible Cas9 enzymes have been developed whose activities are post-translationally regulated with small molecules (Fig. 2; Davis et al., 2015; Liu et al., 2016a). These small molecule-responsive systems use the human estrogen receptor ligand-binding domain (ERT) fused to Cas9 to trigger gene editing events in the presence of the ERT ligand 4-hydroxytamoxifen (4-HT). Davis and coworkers introduced a 4-HT-inducible Cas9 nuclease whose enzymatic activity was inhibited by a strategically placed, self-splicing intein (Intein-Cas9; Davis et al., 2015). Intein-Cas9 was engineered such that its enzyme activity would only be restored after administration of 4-HT, which activates intein-protein self-splicing and permits Cas9’s adoption of a catalytically active form. A related 4-HT inducible Cas9 enzyme was introduced in 2016, dubbed “iCas.” However, this system departs from its predecessor by employing ERT2 as a subcellular carrier versus a covalent inhibitor. As the ERT2 ligand binding domain permits translocation into the nucleus when bound by 4-HT, fusing multiple copies of the ERT2 domain to Cas9 enables bidirectionally regulable genome editing in human cells. Both of these systems demonstrated improved editing specificities over wild-type Cas9, although iCas9 exhibited lower background activity and higher on-target editing when benchmarked against intein-Cas9. While intein-Cas9 and iCas9 show promise for studying dynamic processes in the brain, to our knowledge, they have yet to see use in such experiments.
Advances in photoinducible protein biology have culminated in the development of systems that can control gene-editing and transcription with blue-light irradiation (Fig. 2; Nihongaki et al., 2015; Polstein and Gersbach, 2015). Nihonkagi and coworkers achieved photoinducible gene editing by conjugating fragments of a Cas9 nuclease to protein elements of a dimerizing, light responsive system dubbed “magnets” (Kawano et al., 2015). The fungal-derived magnet system consists of two photoinducible protein elements termed “positive magnet” (pMag) and “negative magnet” (nMag), which are named on the basis of their electrostatic properties (Kawano et al., 2015). This system demonstrated that gene editing could be bidirectionally regulated by light irradiation, albeit with modest indel frequencies and a relatively slow response time (maximal editing ∼48 h). As these limitations may limit paCas9’s usefulness in vivo, additional engineering and optimization are likely required before this technology can be robustly applied in animal studies. While light inducible and optogenetic technologies are widely used in neuroscience research, photoactivatable gene-editors have yet to be applied to the nervous system.
Genomic Regulation with Nuclease-Deficient Cas9
Cas9’s capabilities have expanded beyond conventional genome editing by adapting the system into a programmable DNA-binding module (Fig. 3). To achieve this, Cas9’s catalytic activity was abolished by introducing point mutations into the RuvC1 (D10A) and HNH (H840A) domains to generate nuclease deficient or dCas9. Catalytically-inactive Cas9 retains DNA-binding capability with no apparent loss of targeting or binding specificity (Qi et al., 2013). As discussed below, dCas9-effector fusions provide seemingly endless applications for non-mutagenic genome modification, including transcriptional regulation, epigenome editing, cellular imaging, and RNA interference (RNAi).
Transcriptional and epigenetic regulation with CRISPR-Cas transcriptional control can be achieved by fusing various transcription regulating enzymes to catalytically inactive Cas9 (dCas9). The CRISPR-based activators SunTag, VPR, SAM, and SPH employ various architectures to recruit transcription activating molecules. A, VPR deploys traditional peptide linkers to fuse the tripartite VP64, p65, and Rta effector to dCas9. B, The synergistic activator mediator (SAM) uses the MS2 RNA aptamer to recruit MCPs fused to a p65-HSF1 domain to induce transcription. C, The SunTag system utilizes the a GCN4-epitope array to localize VP64 activators to transcription start sites (TSSs). D, Relatedly, the SPH system uses the SunTag scaffolding array to recruit p65-HSF1 dimers in lieu of VP64. The dCas9-KRAB (E) and the improved dCas9-KRAB-MeCP2 (F) transcriptional repressors use similar strategies inhibit transcription. G, dCas9 fused to the DNA methyltransferase 3A (DNMT3A) enzymatic domain can de-novo methylate CpG dinucleotides in a programmable manner. H, dCas9 fused to ten-eleven translocation’s (TET1) catalytic domain facilitates successive cytosine oxidation and demethylation at methylated CpG sites. dCas9-DNMT3A/TET1 can effectively regulate gene transcription by targeting CpG containing promoter regions for epigenetic modification. dCas9 C-terminally fused to the catalytic core of the human p300 acetyltransferase (p300core) I or histone deacetylase 8 (HDAC8) J can regulate the acetylation status of histone 3 lysine 27 (H3K27) residues to regulate transcription from promoters and both distal and proximal enhancers.
Transcriptional Regulation with dCas9
CRISPR-based transcriptional regulators provide researchers with the ability to assess the functional relevance of specific genes in a variety of neuronal contexts. By manipulating endogenous loci, CRISPR-based overexpression and gene silencing strategies circumvent the shortcomings of cDNA overexpression and RNAi-mediated silencing such as potential protein mislocalization or widespread off-targeting. The first systems endowing activator and repressor capabilities to the CRISPR platform used fusions of tetrameric herpes simplex viral protein 16 (VP64), the NF-κB trans-activating subunit p65 (p65), or the Krüppel-associated box domain of Kox1 (KRAB) to dCas9 (Gilbert et al., 2013; Fig. 3E). When directed to promoter or enhancer sequences, dCas9-VP64 and dCas9-KRAB were capable of inducing or suppressing gene-specific transcription, respectively. These capabilities encouraged their ready adoption for mapping putative cis-regulatory elements in neurodevelopment and neurodegeneration studies (Frank et al., 2015; Heman-Ackah et al., 2016; Huang et al., 2017). Although this first generation of transcriptional regulators could modestly alter transcription, several reports demonstrated that gene expression could be amplified with the provision of multiple sgRNAs per targeted promoter (Gilbert et al., 2013; Maeder et al., 2013; Konermann et al., 2015; Savell et al., 2019). This observation suggested that the overall copy number and enzyme cooperativity of the recruited effectors was responsible for differences in gene expression. Capitalizing on this observation, other groups developed additional CRISPR activator (CRISPRa) and CRISPR interference (CRISPRi) systems with enhanced transcriptional regulatory capabilities (Tanenbaum et al., 2014; Chavez et al., 2015; Konermann et al., 2015). These second-generation systems employ diverse scaffold architectures to recruit transcriptional regulators and maximize effector potency and recruitment.
Early second-generation systems employed an epitope-based scaffolding strategy to increase activator recruitment known as SUperNova (SunTag; Fig. 3C; Tanenbaum et al., 2014). The SunTag scaffold is a peptide array composed of tandem repeating GCN4 epitopes. Transcriptional regulators conjugated to short-chain variable fragments (scFv) with high affinity for the GCN4 epitope can effectively bind the SunTag scaffold, facilitating the formation of multimeric regulatory structures at targeted DNA sequences. Essentially the system is designed to recruit many VP64 transcriptional activation domains to the promoter to enhance transcriptional activation. Indeed, expressing dCas9-SunTag with scFv-bound VP64 activators dramatically increased targeted gene expression compared to that achieved by dCas9-VP64.
Another study (Konermann et al., 2015) examined the regulatory potential of sgRNAs designed to recruit transcriptional activators using RNA aptamers (Fig. 3B). Analysis of sgRNA secondary structures identified regions that were non-interacting with the Cas9 endonuclease and found that mutating distal base pairs in these regions had no influence on DNA binding or cleavage. By substituting sgRNA stem loops with MS2 aptamers that could recruit MS2 coat proteins (MCPs) fused to p65 and heat shock factor 1 (HSF1), it was determined that dCas9-VP64 could upregulate transcription at significantly higher levels when co-expressed with RNA aptamer-containing sgRNAs versus standard sgRNAs.
A separate group screened putative activator domains for gene activation potency, identifying VP64, p65 and the Epstein–Barr virus R transactivator (Rta) as the most potent transcriptional activators. However, dCas9-p65 and dCas9-Rta both exhibited lower transcription rates than the original dCas9-VP64 chimera. To overcome this, combinations of activators were fused with the aim of cooperatively inducing higher gene expression (Chavez et al., 2015). Using dCas9-VP64 as a starting framework, a tripartite fusion of VP64-p65-Rta (VPR; Fig. 3A) was tethered to dCas9 and subsequently assayed for induction capacity, which revealed that gene expression was upregulated between 22- and 320-fold when compared with dCas9-VP64.
Second-generation activators were screened for maximal induction of Ascl1 and Neurod1 genes in HEK293T cells, revealing SAM (Konermann et al., 2015), SunTag (Tanenbaum et al., 2014), and VPR (Chavez et al., 2015) as the most potent gene activators. Subsequent assays revealed SAM as the most consistent in activating high levels of gene expression. Notably, the increased transcription of several tested genes reached orders of magnitude above that induced by dCas9-VP64 (Chavez et al., 2016).
While newly developed CRISPRa systems undergo validation in several common cell types, few have seen any use in neuronal contexts. Savell and coworkers have recently introduced LV vectors capable of robust neuronal VPR expression in vitro and in vivo (in vivo discussion continued below; Savell et al., 2019). Gene overexpression assays in primary cultured neurons demonstrated VPR’s ability to robustly overexpress single or multiple genes with high specificity. Notably, multiplexed gene activation with VPR recapitulated earlier reports of sgRNA-dose responsiveness. Effective activation was demonstrated using an individual sgRNA and significantly increased with the provision of multiple unique sgRNAs.
In contrast to transcriptional activators, few dCas9 repressors capable of enhanced transcriptional downregulation have been developed. Recognizing this deficit, Yeo and coworkers proceeded to perform a similar screen to identify dCas9-repressors capable of robustly inhibiting gene expression (Yeo et al., 2018). Of the screened transcriptional repressors, the bipartite dCas9-KRAB-MeCP2 fusion emerged as the most potent (Fig. 3F).
Regulating Transcription In Vivo with dCas9
Ectopic gene overexpression mediated through viral vector delivery is a popular strategy to investigate neuronal gene regulation (Lentz et al., 2012; Haggerty et al., 2020). As previously described, numerous CRISPRa systems have been developed, enabling the potent activation of multiple genes in various tissues types. However, until recently, these technologies have been limited to in vitro applications because of the difficulty associated with the efficient delivery of multiple large transgenes in vivo. Recently, elements of the SAM and SunTag system were combined to develop a new dCas9-based transcriptional activator, dCas9-SunTag-p65-HSF1 (SPH; Fig. 3D), for in vivo gene regulation (Zhou et al., 2018a). To develop the SPH platform, the VP64 tetramers in the SunTag system were replaced with the p65-HSF1 domains from the SAM system. When combined, these components potently induced gene expression, surpassing the SunTag, VPR, and SAM activators.
In order to circumvent the difficulties associated with viral delivery of large, multicomponent systems to the nervous system, the authors generated a transgenic mouse line harboring a Cre-dependent SPH system. Considering that cell-type and circuit-specific multiplex strategies will likely be required to successfully interrogate gene networks in vivo, Zhou and coworkers performed feasibility experiments on SPH’s multiplex gene activation capabilities. Using a combination of AAV vectors encoding Cre recombinase (hSyn-Cre and CamKIIα-Cre) and sgRNA arrays targeting multiple genes (eight coding genes and two long noncoding RNAs), Zhou and coworkers successfully overexpressed several targeted genes simultaneously. When coupled with existing genome wide CRISPRa sgRNA libraries, these SPH mice provide a useful tool for endogenous gene overexpression and genome wide screening in the brain.
Savell and colleagues sought to optimize the previously developed dCas9-VPR activator for behavioral neuroscience by developing neuron-optimized viral vectors capable of potent, multiplexed gene expression in vivo. By examining VPR expression under the control of several promoters, they were able to identify and produce a LV system that permitted robust VPR expression in vitro and in vivo under the control of the neuron-specific Synapsin promoter. This neuron-optimized LV VPR system was applied in various neuronal contexts and was notably capable of potent, isoform-specific induction of various BDNF transcripts in vivo (Savell et al., 2019).
Until recently, RNAi and conditional Cre-loxP systems have been the predominate methods used for gene knock-down and KO, respectively. However, evidence documenting the significant off-target effects of short hairpin RNA (shRNA) and small interfering RNA (siRNA) has accumulated (Castanotto and Rossi, 2009; Jackson and Linsley, 2010; de Solis et al., 2015). Alternative methods for gene knock-down such as CRISPR-based repressors have been proposed, due to their ability to potently silence gene expression within various contexts. However, the application of CRISPRi technology in neurons has seen limited use in vivo.
Recently, a LV-based CRISPRi system was developed for use in the mammalian brain (Fig. 3E). Using the dCas9-KRAB repressor, synaptotagmin I (Syt1), vesicle associated membrane protein I (Vamp1), syntaxin 1A (Stx1a), and synaptosome associated protein 25 (Snap25), genes responsible for vesicular neurotransmitter release, were targeted in cultured hippocampal neurons. To compare the efficiency of CRISPRi and RNAi-mediated knock-down, sgRNAs and shRNAs were tested for each target gene. CRISPRi produced ∼90% reduction in mRNA and protein levels of all genes targeted, compared to a modest reduction produced by RNAi. Additionally, whole-cell patch-clamp recordings of CRISPRi-targeted hippocampal neurons revealed significant reductions in EPSCs, as expected from disruption of the neurotransmitter release pathway (Zheng et al., 2018).
Numerous studies have reported the potential for Cas9 endonucleases to bind off-target sites (Kuscu et al., 2014; Wu et al., 2014). This, coupled with the observed potency of the dCas9-KRAB repressor, raises concerns for severe off-target silencing. The authors used a “pseudo-target fishing strategy” to determine the frequency of off-targets by expressing dCas9-KRAB with sgRNAs containing unique mismatches with the Syt1 locus. This strategy revealed that Syt1 expression levels remain largely unchanged, indicating that mismatched sgRNAs were incapable of efficiently directing dCas9-KRAB to the Syt1 locus (Zheng et al., 2018).
As cell-type specificity is essential for the interrogation of gene and cell function in the brain, the dCas9-KRAB repression system was modified to restrict targeting to glutamatergic (CaMKIIα-dCas9-KRAB) or GABAergic (VGAT-dCas9-KRAB) neurons. LV infusion into the dentate gyrus revealed a roughly 20% transduction rate of neurons confined to the granule layer. Analysis of dCas9-KRAB+ DG neurons revealed that Syt1 expression was completely abolished in a cell-type specific manner. Likewise, whole-cell patch-clamp revealed that EPSCs within CaMKIIα-expressing neurons were almost completely abolished, with a similar reduction in GABAergic neuron IPSCs (Zheng et al., 2018).
Targeting Syt1 within glutamatergic and GABAergic neurons enables altering of the inhibitory-excitatory (I-E) ratio within the hippocampus. As the hippocampus is implicated in various forms of learning and memory (LaBar and Cabeza, 2006), the authors subjected mice to multiple spatial and associative learning tasks after CRISPRi mediated I-E shifting. Animals receiving CaMKIIα-dCas9-KRAB (shift towards inhibition) exhibited significant performance reductions in spatial memory related tasks (Morris water maze, Barnes Maze, T maze) compared with animals receiving VGAT-dCas9-KRAB (shift towards excitation). In tests of associative memory (fear conditioning), CaMKIIα driving animals demonstrated reduced freezing levels in response to a cued stimulus (tone) in contrast to VGAT driving animals which exhibited slightly enhanced freezing, illustrating that alterations of the I-E ratio within the hippocampus could bidirectionally regulate spatial and contextual fear memory (Zheng et al., 2018).
CRISPR-Based Epigenome Editors
DNA methylation is vitally involved in neurodevelopment and in dynamic gene regulation across various networks in the CNS (Smith and Meissner, 2013). Cytosine methylation within promoter regions permits the controlled regulation of various processes ranging from basic gene transcription to higher-order functions such as learning, memory and cognition. Historically, epigenetic studies have been incapable of determining the functional relevance of specific methylation events due to the limitations of the methylation-inhibiting small molecules 5-azacytidine and 5-aza-2′-deoxycytidine (Heerboth et al., 2014). Although these compounds could be locally injected to induce regional CpG hypomethylation, these shortcomings are largely prohibitive for the precise investigation of disorders such as Angelman’s, Fragile X, Rett syndrome, and Prader–Willi syndrome, all which exhibit significant neurologic phenotypes and aberrant CpG methylation (Butler, 2009). Recent advances in epigenome engineering technology have produced CRISPR-based epigenome editors that couple the programmable targeting of CRISPR with enzymes involved in the DNA methylation pathway (Fig. 3G–J; Liu et al., 2016b, 2018a; Lei et al., 2017).
As dynamic DNA methylation has been proposed to regulate activity-dependent gene expression, Liu and coworkers sought to determine whether their LV dCas9-TET1 system could induce brain-derived neurotrophic factor (BDNF) expression by targeting the BDNF IV promoter for demethylation in cultured primary neurons (Fig. 3H; Liu et al., 2016b). Neuronal dCas9-TET1 expression successfully increased BDNF expression 6-fold. However, “no sgRNA” controls also produced a nearly 2-fold increase in BDNF expression, demonstrating this system’s potential for non-specific gene induction. CRISPR-epigenome editors have also been used preclinically for therapeutic studies. For example, dCas9-TET1 was used to demethylate the CGG trinucleotide expansion in the 5′ UTR of the Fmr1 gene in models of fragile X syndrome (FXS; Persico and Napolioni, 2013; Liu et al., 2018a). dCas9-TET1 targeting to the Fmr1 5′ UTR in in vitro-derived FXS neurons significantly reduced CGG trinucleotide hypermethylation and the associated hyperexcitable phenotype. Remarkably, dCas9-TET1-treated inducible pluripotent stem cell (iPSC)-induced FXS neurons retained high levels of FMRP expression months after their engraftment into live mouse brains.
Beyond the transcriptional regulation mediated by dynamic DNA methylation, histone modifications gatekeep gene expression by altering chromatin conformation and the accessibility of cis-regulatory elements to DNA binding proteins (Yarrington et al., 2018). CRISPR-based epigenome editors have been used to uncover the functional importance of discrete regulatory elements (Hilton et al., 2015; Chen et al., 2019). Using dCas9-p300 and dCas9-HDAC8 (Fig. 3I,J), the histone modifications at the second enhancer (Enh2) of the neuronal immediate early gene (IEG) Fos were shown to fine tune the degree of activity-induced transcription. In other words, the type of histone modification installed by p300/HDAC8 could slightly increase or decrease activity-dependent Fos transcription. However, inducing a heterochromatic state with HDAC8 could not completely silence Fos activity, and inducing a euchromatic or “protranscriptional” state was insufficient to induce Fos transcription without neuronal activity. This observation contrasts the constitutive gene activation mediated by other CRISPRa systems, which if targeted to Enh2, would presumably induce Fos without neuronal activity. The effectiveness of CRISPR-based epigenome editors highlights the potential for these new tools to elucidate the functional relevance of non-coding and epigenetically regulated elements to animal behavior, neuronal function and disease.
Engineering the Neuronal Transcriptome with RNA-Targeting CRISPR Effectors
Programmable DNA-targeting Cas9 nucleases have been used for in vivo gene studies. However, tools enabling the study of RNA function are severely lacking. Recently, the diverse group of Class 2 CRISPR-Cas systems has been expanded to include the Type VI, RNA-targeting Cas13 family of effectors (Abudayyeh et al., 2016, 2017). Despite their recency, RNA-targeting CRISPR systems have been engineered for targetable RNA visualization, knock-down, base-editing, and in vitro isoform manipulation (Figs. 4, ,5;5; Abudayyeh et al., 2017; Cox et al., 2017; Konermann et al., 2018). The Cas13 family of endonucleases are characterized by a single-effector protein containing two higher eukaryotes and prokaryotes nucleotide-binding (HEPN) ribonuclease (RNAse) domains (Abudayyeh et al., 2016). Unlike their DNA-targeting counterparts, Cas13 effectors do not require tracrRNAs for pre-crRNA processing, nor do they require PAM sequences for nucleic acid targeting and non-self-recognition. Instead, sequences that are enriched proximally to protospacer targeting sites are referred to as protospacer flanking sequences (PFSs). Notably, several Cas13 variants have been shown to not require a PFS for RNA cleavage (Cox et al., 2017). Multiple studies have reported a large amount of divergence amongst the Type VI family, often reporting little sequence conservation among Cas13 nucleases other than the characteristic HEPN RNase domains; for a more complete discussion of their individual properties we suggest reviewing (Shmakov et al., 2017).
RNA targeting with CasRx. (A) CasRx can efficiently target and cleave RNA via its dual HEPN nuclease domains. Unlike DNA targeting Cas9 endonucleases, several Cas13 orthologues do not exhibit PFS (PAM site analogue) requirements. Mutating HEPN catalytic residues (R295A, H300A, R849A, H854A) preserves CasRx’s RNA binding ability, allowing CasRx to be adapted for fusion constructs. (B) Splice isoform engineering|decatalyzed CasRx (dCasRx or dCas13d) fused to the splicing factor hnRNP1 can be targeted to various splice elements (splice acceptors, splice donors, intronic branch points, etc.) to induce exon skipping and isoform selection.
Base editors. A, Adenine base editor (ABE) and cytosine base editor (CBE) catalyze the deamination and alteration of DNA nucleobases via chimeric Cas9n-DNA deaminase fusions. Nicking (single strand DNA cleavage) of the non-edited strand increases base-editing efficiency by inducing cells to repair the cleaved strand using the edited strand as a template. B, The Cas13-based RNA base editor RNA-editing for programmable A to I replacement (REPAIR) mediates the conversion of adenosine to inosine, while RNA editing for specific C to U exchange (RESCUE) mediates the conversion of cytosine to uracil.
Numerous studies have compared the knock-down ability of multiple Cas13 subtypes and orthologues to RNAi, which have overwhelmingly demonstrated that Cas13’s RNA knock-down capabilities are superior to those of shRNAs (Abudayyeh et al., 2017; Cox et al., 2017; Konermann et al., 2018). Additionally, the recently discovered Cas13d effector, Ruminococcus flavefaciens-Cas13d (CasRx; Fig. 4A), has been shown to more effectively silence gene expression than other well-established methods such as CRISPRi (Konermann et al., 2018). When targeted to the endogenous B4GALNT1, ANXA4, and HOTTIP genes in HEK293FT cells, CasRx demonstrated a remarkable median knock-down efficiency of 96% compared with 53% knock-down produced with sequence-matched shRNAs. Furthermore, CasRx did not generate any detectable off-target transcriptional changes, which starkly contrasts shRNA-induced silencing of an excess of 500–900 off-target genes (Konermann et al., 2018). CasRx also outperformed CRISPRi (dCas9-KRAB) mediated repression, which produced a median 53% knock-down rate when targeted to the same genes. Other recently described Cas13 subtypes have been shown to robustly knock-down RNA in mammalian cells. Compared to Cas13a (LwaCas13a-msfGFP-NLS; Abudayyeh et al., 2017) and Cas13b (PspCas13b-NES; Cox et al., 2017), CasRx demonstrated greater transcript knock-down ability (median knock-down rates; Cas13a, 80%; Cas13b, 66%; CasRx, 97%). Remarkably, of 14 sgRNAs targeted to both coding and non-coding RNA, CasRx yielded at least ∼80% transcript knock-down, suggesting that CasRx could be used to regulate any RNA in the cell.
Several degenerative diseases have been linked to mutations within individual pre-mRNA elements. For instance, mutations within exons 45–55 or exon 23 of the Dystrophin gene produce the muscle degeneration associated with Duchenne muscular dystrophy (DMD; Ousterout et al., 2015; Long et al., 2016). Likewise, neurodegenerative tauopathies such as Frontotemporal dementia with parkinsonism linked to chromosome 17 (FTDP-17) is associated with point mutations in exon 10 of the MAPT gene, which determines which Tau protein isoform is expressed in neurons (Boeve and Hutton, 2008). As previous studies have reported success in models of DMD using exon-skipping strategies (Nelson et al., 2019), Konermann and coworkers tested whether dCasRx could efficiently drive isoform selection by developing a dCasRx-RNA splice effector fusion (Fig. 4B).
Pre-mRNA splicing is mediated by interactions between cis-acting elements (splice acceptor/donor sites, intronic branchpoint nucleotides, etc.) and the trans-acting spliceosome (Matera and Wang, 2014). Within the cohort of pre-mRNA interacting molecules are the heterogeneous nuclear RNPs (hnRNPs), a ubiquitously expressed group of splice factors that facilitate alternative splicing by inhibiting exon exclusion (Wang et al., 2015). The hnRNPa1-CTD was fused to dCasRx and targeted to several putative splicing elements, which successfully induced exon-skipping in a fluorescence splicing reporter. In order to determine whether skipping exon 10 of the MAPT gene could decrease the accumulation of pathogenic (isoform 4R) tau, cortical neurons differentiated from patient-derived iPSCs were transduced with AAV encoding dCasRx-hnRNPa1 and three exon 10 targeting sgRNAs. dCasRx-hnRNPa1 mediated exon-skipping was shown to reduce 4R/3R ratios by 50%, a level similar to unaffected controls (Konermann et al., 2018).
These results demonstrate the ability of Type VI, RNA-targeting Cas13 effectors for enhanced RNAi and manipulation. In the past, applications of dCas13 effector fusions have been limited by their large size. Therefore, CasRx’s short coding sequence (∼2.9 kb) makes it highly suited for use in AAV vectors. As described above, the CasRx fusion and three sgRNAs fell below AAV packaging limitations, a characteristic that may inspire the future development of CasRx-based effectors that are capable of elucidating RNA function in the brain.
Base and Prime Editing
Existing CRISPR technologies equip researchers with a powerful, multifunctional platform to investigate a staggering number of biological questions, however these tools are not without drawbacks. DSBs created by Cas9 nucleases often result in haphazard DNA repair and indel formation, which can frequently produce extensive sequence heterogeneity and yield several unwanted or deleterious DNA products. Technologies have been developed that circumvent problematic DSBs and imprecise cellular DNA repair processes through the use of enzymes (Fig. 5) that can alter RNA and DNA nucleotides in situ, or more recently, prime editors that can faithfully install edits through reverse-transcription of an RNA template (Fig. 6). These technologies, termed base editors, rely on dCas9 fusions to nucleobase deaminases to directly install point mutations without the need for DSBs. Existing base editors are collectively able to catalyze all possible transition mutations (C to T and A to G, point mutations) in DNA, with recent developments in RNA base editing allowing the conversion of A to I, and C to U bases as well. DNA and RNA base editors are extensively discussed in Rees and Liu (2018). As of yet, no studies have reported the use of base editors in any neuronal context. However, the growing number of single nucleotide polymorphisms (SNPs) implicated in psychiatric and neurologic diseases and the finding that the mRNAs of various neuronal ion channels and synaptic receptors undergo RNA editing may prompt the future use of these tools in neuroscience laboratories (Behm and Öhman, 2016).
Prime editing. Prime editors (PEs) use a partially decatalyzed Cas9(H840A) nickase, a prime-editing RNA (pegRNA) and an engineered reverse transcriptase (RT) to precisely introduce DNA edits; pegRNAs contain a primer binding site (PBS) which anneals to the nicked target strand, allowing sequence extension through reverse transcription and production of the edited strand. pegRNA-PBS reverse transcription produces an edit-containing 3′ flap and an unedited 5′ flap which undergoes preferential degradation by endogenous 5’−3′ exonucleases. The remaining edited 3′ flap anneals and is ligated, resulting in a mismatched heteroduplex which can be resolved by cellular DNA repair pathways. Targeting the unedited strand with a separate sgRNA increases editing efficiency and stimulates preferential DNA repair to permanently install edited DNA.
Prime editors present the latest advance in precision gene editing. Anzalone and coworkers introduced a Cas9-nickase (Cas9n)-based system that couples the DSB-free editing strategy pioneered with base-editors to an sgRNA-based RNA donor template (Fig. 6; Anzalone et al., 2019), a strategy similar to one recently introduced in yeast (Sharon et al., 2018). Prime editors are multicomponent systems comprised of a chimeric Cas9n-reverse transcriptase and a prime editing guide RNA (pegRNA). Both the target locus and the desired DNA edit are encoded on the pegRNA, which harbors the standard Cas9 sgRNA components and a 3′ extended RNA template. Cas9n cleavage of the PAM-containing strand allows donor-template invasion and hybridization, which permits RNA-template reverse transcription and effective installation of the desired edit. This prime editing strategy was shown to successfully introduce broad classes of edits with lower indel frequencies than Cas9-mediated HDR in multiple cell types in vitro, including a modest editing frequency (6–8%) in primary neuronal cultures. Although a promising development, the frequency of genome-wide off targets and unintended reverse transcription products remain unknown. This, in concert with the modest editing frequency achieved with the latest prime editor, may preclude its immediate use in vivo. Nonetheless, this technology presents an exciting new development towards achieving high-fidelity, corrective gene editing with CRISPR.
CRISPR Screens
The recent exponential advances in NGS technologies and the easy design and production of large numbers of unique sgRNAs has facilitated the high-throughput investigation of various psychiatric and neurodegenerative disorders, cancer, and essential gene functions through large-scale CRISPR screens (Fig. 7). CRISPR-mediated screens combine high-throughput, single-cell sequencing technologies with genome-wide sgRNA targeting libraries optimized for gene KO (Sanjana et al., 2014; Doench et al., 2016; Morgens et al., 2017; Wang et al., 2018; Liu et al., 2019), activation (Horlbeck et al., 2016; Joung et al., 2017; Chong et al., 2018; Liu et al., 2018b; Sanson et al., 2018), and silencing (Horlbeck et al., 2016; Liu et al., 2017; Sanson et al., 2018) applications. Recent applications of CRISPR-screening have produced new experimental pipelines that permit the unambiguous contribution of risk-associated genes to disease phenotypes (Thyme et al., 2019) and the determination of cellular-lineage and heredity in developmental studies (McKenna et al., 2016; Raj et al., 2018). For example, CRISPR-Cas9 was recently used to perform a mutant-phenotyping screen on schizophrenia-associated genes identified in human genome-wide association studies (GWAS; Thyme et al., 2019). Cas9 was used to mutagenize several risk-associated genes in developing zebrafish. These mutants were then subjected to behavioral and structural analysis which allowed Thyme and coworkers to successfully uncover phenotypes for multiple understudied genes. A separate zebrafish study deployed a large-scale CRISPR-Cas9 technique (GESTALT; see McKenna et al., 2016 for additional background) combined with single-cell RNA-seq (scRNA-Seq) to determine cellular fate and lineage characteristics in developing brains. Paired with an inducible Cas9, DNA barcodes harboring specific target sequences were used to indicate whether DNA editing occurred in a specific cell; because genomic bar code expression results in cellular progeny with identical barcode sequences, this allowed Raj and coworkers to determine the lineage histories for a plethora of cell types in the developing zebrafish brain.
High-throughput genetic screening with CRISPR. Large scale genetic screens can be performed in iPSCs expressing CRISPR machinery. Transduction of iPSCs with pooled LV sgRNA libraries permits the selection and expansion of construct-positive cells before in vitro neuronal differentiation. CRISPR-KO, CRISPRi, and CRISPRa can be coupled with single cell and NGS technologies for genome-wide or targeted gain- and loss-of function screens.
While most screens are performed in vitro or ex vivo, two CRISPR-Cas9 mediated in vivo screens have recently been reported in the brain (Chow et al., 2017; Jin et al., 2019). A recent in vivo screen (Perturb-Seq, first described by Dixit et al., 2016) aimed at systematically uncovering the phenotypes of a large panel of ASD-related genes was performed by coupling cell-type specific transcriptomics and a lentivirus-mediated sgRNA library targeting 35 putative ASD-risk genes. Ventricularly injecting LV-sgRNA libraries into developing embryos in utero permitted postnatal, single-cell transcriptional profiling and identification of multiple gene clusters from cortical and striatal tissue. CRISPR knock out coupled with a scRNA-seq readout readily enabled differential gene identification, subsequent perturbation and phenotyping for a number of ASD-risk genes involved in distinct molecular pathways across variant cell types.
The earliest reported in vivo screen was directed at investigating the functional and tumorigenic consequences of significantly mutated genes (SMGs) that were previously identified in tumor samples taken from human glioblastoma multiforme (GBM) patients (Chow et al., 2017). A pooled AAV-sgRNA library [mouse homolog tumor suppressor library (mSTG)] targeting various risk-genes was hippocampally or ventricularly infused into mice, which produced GBM-characteristic tumor growth at four months postinjection. Histologic, transcriptomic and genetic characterization of AAV-CRISPR-mediated GBM tumors, in vivo and ex vivo, permitted the successful identification and correlation of single and co-occurring tumor drivers to GBM mutations identified in human patients.
Although CRISPR-based screens are heavily used in oncology research (Hart et al., 2015; Tzelepis et al., 2016; Chow et al., 2017), these tools have garnered significantly less attention for large-scale genetic studies in disease-relevant cell types such as differentiated neurons. Tian and coworkers recently performed several CRISPRi screens to elucidate functional contributions of various genes to cell survival, differentiation, transcriptional regulation and morphology in human iPSC (hiPSC)-derived neurons (Tian et al., 2019). In an initial survival screen, dCas9-BFP-KRAB and the LV H1 sgRNA library were used to target ∼2300 genes comprising the “druggable genome.” CRISPRi-mediated gene knock-down uncovered a strong neuronal dependence on sterol/cholesterol metabolism genes and enhanced neuron survival when members of the integrated stress response (DLK, JNK, PERK) were knocked down. Tian and coworkers also performed screens that identified common regulators of variant transcriptional programs in iPSCs and neurons, as well as several genes that contributed to neuronal longevity and morphology.
Existing Challenges for CRISPR Gene Editing
Despite the explosive progress of CRISPR-mediated genome engineering in the last decade, significant challenges for clinical and preclinical applications remain. For example, concerns regarding CRISPR’s immunogenicity, targeting efficiency, fidelity and optimal delivery will need to be addressed before CRISPR can fulfil its clinical and research potential.
CRISPR delivery in vivo has been accomplished virally, with RNPs and nanoparticles. For preclinical studies using small animals, these delivery methods are sufficient, since experiments can be conducted where useful data can be generated by targeting relatively small body areas. Viruses and RNPs can deliver their necessary cargo to regions of this size reasonably well. However, improvements can be made to increase the ease of delivery and the area of tissue that could be effectively targeted with CRISPR systems. For example, conventional AAVs and LVs need to be stereotaxically injected intracranially to gain access to the brain and usually will not transduce more than a few cubic millimeters of tissue. More recently, AAVs with modified capsids have been developed that can cross the blood brain barrier, so they can be administered systemically and transduce brain cells (Chan et al., 2017). Although these developments are encouraging, they still need more development for clinical utility. Notably, non-human primate research and clinical human studies will generally benefit from less invasive routes of delivery that can target larger regions of the brain. This will be an important hurdle to overcome if CRISPR is to ever realize its full potential of treating CNS diseases.
For any gene modification technique, its specificity and accuracy are paramount, especially for clinical applications. While high-fidelity SpCas9 variants have been developed (Kleinstiver et al., 2016; Slaymaker et al., 2016; Chen et al., 2017; Casini et al., 2018; Chatterjee et al., 2018; Hu et al., 2018), the off-targeting frequencies and loci for therapeutic sgRNAs will need to be thoroughly characterized in vitro before use in human therapies. For gene KO in preclinical applications, SpCas9’s fidelity is likely sufficient, especially since researchers can perform independent experiments with differing sgRNAs designed to KO out their intended gene. Given that differing sgRNAs would likely not exhibit the same off targets, if the same phenotype is obtained with both sgRNAs, then their result would likely be due to the KO of the intended target.
Preclinical studies using CRISPR-Cas have generated significant enthusiasm for the future of personalized gene therapies. However, as CRISPR becomes implemented clinically, aspects of its safety for use in human therapies have received extensive scrutiny. Recently, various preclinical studies have described the immunogenicity of CRISPR nucleases following systemic (IV) administration to laboratory mice (Chew et al., 2016; Nelson et al., 2019). Host anti-vector and transgene responses are discussed elsewhere (Sun et al., 2003; Rabinowitz et al., 2019; Wang et al., 2019). Additionally, preexisting adaptive immunity against S. pyogenes and Staphylococcus aureus Cas9 have also been reported in humans (Charlesworth et al., 2019). However, these findings are unsurprising given the high frequency at which these bacteria infect humans (Lowy, 1998; Roberts et al., 2012). While SpCas9 and SaCas9 remain two of the most broadly used CRISPR enzymes, new orthologues derived from non-pathogenic bacterial species may be required for human therapies where preexisting immunity is a concern. Alternatives such as orthologue specific-epitope engineering or short term suppression (Chew et al., 2016) may theoretically ameliorate immune responses in the short-term. However, the long-term expression of AAV-mediated therapies and their potential for genome insertion at DSB sites (Miller et al., 2004; Hanlon et al., 2019), may limit the feasibility of immunosuppressive approaches.
The low efficiency of precise editing (corrective editing via HDR, prime editing, etc.), in neurons is another significant hurdle for the use of CRISPR for neuroscience research and human therapy. The available data indicate that precise editing occurs at relatively low levels in neurons, limiting the utility of these methods and currently making them unlikely to have any benefit clinically. Although precise editing occurs relatively infrequently in most cell types compared to NHEJ-mediated indel formation, disorders that afflict mitotically active cell populations may be more amenable to HDR-based therapies. For example, hemopoietic progenitor cells can be genetically modified ex vivo, clonally selected efficiency, expanded and then re-implanted, essentially bypassing the issue of inefficient HDR mediated precision editing. In another example it was recently demonstrated that AAV-CRISPR could be delivered via tail injection in a mouse model of hereditary tyrosinemia Type 1 (HTI) to correct genetic mutations within the liver (Yin et al., 2014). Although only a small percentage of hepatocytes (<1%) harbored the therapeutic edit, the gene correction provided a fitness advantage to edited cells, which allowed them to repopulate the liver. Unfortunately, given that neurons are postmitotic, precise, CRISPR-mediated editing has limited utility for the foreseeable future until methods are developed to increase its efficiency.
Conclusions and Future Directions
The CRISPR-Cas system has emerged as a highly adaptable platform with extensive utility in multiple areas of biomedical and basic science. Given its ability to target nearly any gene or RNA transcript, alter gene expression and modify epigenetic states with high specificity, CRISPR-Cas represents an invaluable tool helping drive the rapid pace of discovery in biological sciences. While early studies only demonstrated its use in peripheral tissues, recent efforts have produced CRISPR-Cas systems amenable for use in the CNS. Additionally, the development of CRISPR-expressing animals, as well as the discovery of AAV-compatible orthologues, have provided substantial tools for probing neuronal function at multiple levels of analysis. While newly developed CRISPR-transgenics may be crossed with existing Cre-driving lines, novel CNS-optimized tools will likely require viral vector encoding and delivery. Challenges associated with viral vectors such as packaging constraints, low virus infectivity and low gene editing efficiencies remain limiting factors for using CRISPR in the brain. In order to maximize the therapeutic and research potential of available systems, existing delivery methods must be optimized and new, more effective ways of introducing these systems must be developed. Undoubtedly, future improvements and applications of CRISPR-Cas technology will surface. Despite these challenges, recent advances in CRISPR-Cas technology have provided researchers with powerful new tools for engineering the neuronal genome.
spacer
Can CRISPR bring back extinct animals?
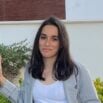
The concept of de-extinction has been around for many years. Once considered a fanciful idea, recent advancements in technology – notably CRISPR – have made bringing back extinct animals such as mammoths an extremely feasible proposition that investors are willing to put their money into, with an aim of reversing and preventing biodiversity loss.
The idea of bringing back extinct animals was first explored in the 20th century through a technique known as back-breeding, which involves the application of selective breeding – similar to that of traditional selective breeding to create an animal with certain desired traits – to resurrect ancestral traits that have been lost over time through evolution.
While back-breeding is still one of the main techniques used, the first ‘successful’ de-extinction came via cloning. In 2003, scientists used a cloning technique adapted from Dolly the Sheep – the world’s first ever mammal cloned from an adult somatic cell – to create a clone of the last living bucardo, named Celia, who died in 2000.
Now, the newest method being explored for de-extinction is the gene editing technology known as CRISPR – specifically CRISPR/Cas9 – which is still fairly new to the scene, having first been used as a gene-editing tool in 2012. Not only is CRISPR viewed as one of the most promising methods for tackling a range of diseases, it also appears to be producing positive results when it comes to de-extinction.
Science behind using CRISPR for de-extinction
CRISPR is an engineered cellular technology that has an RNA guide that is programmed to target specific areas on a genome, with the Cas9 protein acting as scissors. It essentially adds or deletes genetic information, allowing scientists to edit DNA.
The first step in using CRISPR for de-extinction is sequencing the extinct animal’s genome, as this will provide a blueprint for it. Sequencing the genome will determine the order of the four bases, or chemical building blocks (A, C, G, and T), that make up the DNA molecule. Scientists also need to get hold of the genome of its closest living relative in order to achieve this.
“If you know the sequence of the genome of your extinct animal, and if you have the genome of its relative, you can look for the differences and just use CRISPR to edit the differences – that’s the basic idea,” said Professor Tom Gilbert, evolutionary biologist at the University of Copenhagen.
To do this, scientists compare the genomes of the extinct animal and its living relative and insert critical parts of the extinct animal’s genome into the genome of its relative. For example, in the case of the mammoth, its cold-resistant traits need to be inserted into the Asian Elephant genome.
However, in reality, it is a lot more complicated than that due to the fact that ancient DNA is very fragmented, having been broken down over time by bacteria and exposure to UV light. The shorter the fragments, the more difficult it becomes.
Once the extinct animal’s crucial traits have been inserted into its relative’s genome, a CRISPR/Cas9 complex containing RNA needs to bind to the identified gene in the genome of the relative and cut into the double strands of its DNA, allowing scientists to eventually create a hybrid version of the extinct animal.
But, as promising as the technology is, using CRISPR for de-extinction does ultimately have its limitations, in the sense that it can’t bring back an exact replica of an extinct species. These limitations were laid out in the Christmas Island rat study in 2022.
“The study was to show how, for various reasons, if you try and bring something back, what you end up with is not really what you lost to start with, it’s some kind of hybrid version, which is fine,” said Gilbert, who co-authored the study.
The truth is that gene editing technology is not advanced enough yet to bring back an exact replica in a timely manner. It would take hundreds of years to change all of the differing sequences to the extinct animal’s DNA.
Gilbert said that he isn’t against de-extinction, but that he wants people to understand that it doesn’t mean bringing back a full version of an extinct animal. “My worry is that if someone gives a company a whole lot of money to bring back a mammoth, and the end product isn’t a mammoth, they won’t be happy, and the last thing the world needs is more people not trusting in science,” he said.
Current CRISPR de-extinction projects: resurrecting the wooly mammoth, the thylacine and the dodo
If you’re looking for proof of the allure de-extinction currently holds on investors, then Colossal Biosciences, which is at the forefront of the current scientific landscape surrounding de-extinction, is the perfect example. It secured $150 million in Series B financing two years after the company’s initial launch in 2021, and is involved in ongoing projects to resurrect the wooly mammoth, the thylacine and the dodo using CRISPR.
The return of the mammoth with CRISPR is Colossal’s landmark project. Given that the species died out around 4,000 years ago, it seemed unlikely it could be brought back. But scientists managed to recover DNA from mammoth fossils frozen in the Arctic tundra, in Siberia, in 2021. Now, Colossal is working on splicing bits of this DNA into the genome of its closest relative, the Asian elephant, which shares 99.6% of its DNA.
Colossal also announced in January 2023 that it has launched a project to bring back the iconic dodo, with the help of University of California Santa Cruz professor and lead palaeogeneticist at the company, Beth Shapiro, who led the team that sequenced the dodo’s genome for the first time in March 2022.
But at this moment in time, it’s the project to de-extinct the lesser-known thylacine – a marsupial apex predator from Australia – that shows the most promise, given it died out less than 100 years ago, meaning its DNA is more accessible and the leftover fragments are longer. The project is led by the Thylacine Integrated Genetic Restoration Research (TIGRR) Lab at the University of Melbourne, who partnered with Colossal last year. Geneticist Professor Andrew Pask, Head of TIGRR, managed to sequence the thylacine’s genome in 2017 using an extremely well-preserved sample.
“Museums Victoria in Australia had one pouch young (pup) that was placed into ethanol when it was collected more than 100 years ago. This was unusual – most go into formalin – but the ethanol preserved the DNA better, making the genome sequencing tractable for this species,” said Pask.
TIGRR also has access to a platinum-level genome for the closest living relative to the thylacine; the fat-tailed dunnart. The two species share 95% of their DNA, meaning the dunnart can provide the cells and genomic blueprint to create a functional thylacine genome.
There is still a long way to go, though. “The sheer scale of the number of edits which need to be made is the bulk of the work here. We also need to develop assisted reproductive technologies (ART) for marsupials; things such as embryo transfers, IVF, stem cells,” said Pask.
However, he stated that the partnership with Colossal will help to advance the thylacine de-extinction quest: “The partnership with Colossal brings together an incredible team of dedicated biologists all working on the same goal – of recreating the thylacine. The DNA editing technologies leveraged by Colossal will greatly accelerate the project.”
A conservation solution or an ethical concern?
Depending on who you talk to, de-extinction can either be viewed as a solution to biodiversity loss and act as a progressive conservation tool, or it can be seen as an unnecessary distraction from current conservation efforts and could have a negative impact on current ecosystems.
The former is the primary reason behind the recent push for de-extinction; it can potentially provide an opportunity to bring back the animals that were driven to extinction by humans – rather than through natural, evolutionary causes – resulting in the removal of key balancing factors within specific ecosystems. In theory, re-introducing certain extinct species to their natural environment will favorably impact biodiversity because of this.
The thylacine was one such species forcibly removed through human acts that played an integral part in its ecosystem. “The thylacine was completely unique among living marsupials. Not only did it have its iconic wolf-like appearance, but it was also our only marsupial apex predator. Apex predators form extremely important parts of the food chain and are often responsible for stabilizing ecosystems,” explained Pask.
In fact, human interference is the root cause of most modern extinctions, which is a trend that is becoming increasingly more noticeable. According to a report released in October 2022, the planet’s wildlife populations have decreased by around 69% since 1970 due to things like rainforest destruction, pollution, overfishing and trophy hunting.
De-extinction could also be used for the conservation of existing species, through the use of freezing and biobanking stem cells, which can then be used to repopulate an area with an endangered species.
However, there are also multiple ethical concerns surrounding de-extinction, with some scientists and conservationists arguing that the habitat of species’ brought back to life will no longer exist, and they might not be able to survive by themselves in the wild, leading to them ending up in cages and zoos. Another major concern is whether they might upset existing ecosystems and alter food chains if they are re-introduced into the wild.
There are also fears de-extinction might undermine conservation efforts for existing animals by diverting funds away from well-known conservation techniques, like the creation of protected areas and the management of small animal populations to help them survive.
Phil Brooke, research and education manager at Compassion in World Farming, said: “De-extinction is not a good solution. It is likely to involve procedures that cause suffering to the animals involved and is unlikely to produce a range of animals with the genetic diversity required to ensure long-term survival. Above all, it is a distraction from the real challenge, which is that we must act quickly to stop even more extinctions of the animals we currently have.”
There is no precedent for bringing an extinct animal back to life for long enough to witness the effect it has on the ecosystem it is re-introduced into, so it’s difficult to say yet whether de-extinction will have an overall positive or negative impact. But, ultimately, scientists hope that CRISPR will prove to be the solution to both de-extinction and the conservation of existing species.
“It is through great partnerships like ours with Colossal that de-extinction will soon become another tool in our conservation tool kit, and one that will be used to restore lost biodiversity and ecosystems – something which is becoming more and more critical with our changing planet,” said Pask.
spacer
847K subscribers
Due to the dramatic shift in climate and other human interventions, there are a lot of plant and animal species that are extinct than those that are alive today. This is actually a shame, especially to the scientist’s community who should have given it all to study these creatures. However due to advances in genetics and cloning, bringing this animals back from extinction seems more of a possibility than ever. Let’s look at some of the top 20 animals scientists are ready to bring back. 1. The dodo 30years after its discovery, the dodo became extinct. It’s only known habitat was Mauritius Island where it had been living peacefully, who knows for how long until in the 1590s when their death showed up. The dodo had no fear of people because it had not seen humans before so it was easy to cab this creature to death. These birds were big and heavy, a little over 3 feet tall and weighing about 39 pounds. Luckily the dodo may soon become un-extinct as scientists just need to collect few DNA samples and implant them into their closest relative, the pigeon. 3. The woolly mammoth Even though most of the woolly mammoths died out about 10,000 years ago, a population of about 500 to 1000 of them was still living on wrangle island up to 4000 years ago. This animal was about the size of an African elephant with a shoulder height of 11 feet and weighed about 6tons.They had a thick double coated far and fluffy ears that helped it survive harsh conditions. Many discovered specimens frozen in the arctic make it possible for scientists to use their DNA and create a high breed embryo of a wooly mammoth and an elephant that has already been nicknamed mammophant. 5. The Siberian unicorn There you go for that’s what a real unicorn looks like, and it was more like a rhino than a gracious horse. Siberian unicorn discovered in Kazakhstan showed that it had just disappeared 29,000 years ago unlike scientists had earlier thought, and that’s great for people who wish to see a unicorn someday, because it was technically a unicorn and not some magical fairy tale type. With one big horn coming out of its skull, it was 15 feet long 6 feet tall with a whopping 9,000 pounds in weight! Mh.. there you go fox. 6. The woolly rhinoceros Alongside the woolly mammoth is the woolly rhinoceros a fluffy extinct animal that scientists want to bring back.This animal recently lived in the arctic snows about 10,000 years ago. This furry rhino is quite famous and often makes an appearances in the french chauvet-point d’ arc cave. The arctic permafrost has done a great job by preserving quite a lot of specimen and now the woolly rhinoceros has the same chances of being resurrected as a mammoth. 7. The saber-toothed tiger Just about the size of your modern day big cat, this extinct animal being brought back by scientists could get up to 13 feet long and weighed from 200 to 600 pounds. It had a muscular neck, powerful front legs and sharp teeth protruding from the upper jaw. Just looking at those teeth, am sure you’re thinking this is extinct animals we shouldn’t bring back to life! These freelance predators disappeared just 11,000 years ago and because their original habitat was pretty cool their fossils have remained intact just like a mammal. 10. The Irish elk Also known as the giant dear, the Irish elk is one of the animals that dint manage to survive the ice age. Despite its extinction about 12,000 years ago, researchers have discovered Irish elk fossils that are only 7000-8000 years old in Siberia. If this is one of the extinct animals scientists want to bring back, well it will be a great sight to see. 11. Pyrenean ibex This I a subspecies of a Spanish ibex that went into extinct for the last 20 years. They were hunted for meat for over 200 years until their exhaustion in the year 2000. But, can scientists bring back extinct animals? Yes, this species was brought back from extinct in 2006, though it went back again in just few minutes due to a deformed respiratory system. 14. The quagga This animal that went extinct in 1983 due to hunting is actually a subspecies of the plain zebra. The two species had the same DNA and shared the same genotype though their observable characteristics are different. This extinct animal scientists are bringing back are almost identical to the extinct quagga and can be released into the wild. 18.The megalania The magalania were part of the megafaunal assemblage that inhabited southern Australia during the Pleistocene. There is a larger initiative though aimed at retrieving the long gone megalania putting it on the list of some of the extinct animals being brought back to life. Which one of them you want to see in real life? Do you think we should bring them back? Let us know in the comments box below. #UltimateFact #Facts #Animals
spacer
Colossal lands $15 million to restore the woolly mammoth to the Arctic by genetically modifying their Asian elephant cousins.

4 min read

You’ve heard of startups building computer chips, delivery drones and social networks. One called Colossal has a very different goal: bring the woolly mammoth back from extinction by 2027 using CRISPR, a revolutionary gene-editing technology.
The plan isn’t to re-create true woolly mammoths but instead to bring their cold-adapted genetic traits, which include small ears and more body fat, to their elephant cousins, creating a hybrid that can wander the tundra where mammoths haven’t been seen for 10,000 years. Colossal’s co-founders are Chief Executive Ben Lamm, who started five companies before this, and George Church, a Harvard Medical School professor with deep CRISPR expertise.
“Our true North Star is a successful restoration of the woolly mammoth, but also its successful rewilding into interbreeding herds in the Arctic,” Lamm said. “We’re now focusing on having our first calves in the next four to six years.” (so three years from now)
It’s an interesting illustration of an imperative sweeping the tech world: Don’t just make money, help the planet, too. Tesla’s mission is to electrify transport to get rid of fossil fuels that hurt Earth. Bolt Threads wants to replace leather with a fungal fiber-based equivalent that’s easier on the environment than animal agriculture. Colossal hopes its work will draw attention to biodiversity problems and ultimately help fix them.
Artificial wombs and other technology spinoffs
Church said he expects spinoffs from the company’s biotechnology and genetics work.
“The pipeline of large scale genome engineering techniques can be applied to many other applications beyond de-extinction, and therefore [are] most promising for commercialization,” he said.
One technology ripe for commercialization is multiplex genome engineering, a technique Church helped develop that speeds genetic editing by making multiple changes to DNA at once.
Colossal also hopes to develop artificial wombs to grow its mammoth embryos. Just growing 10 woolly mammoths with surrogate elephant mothers isn’t enough to get to the large-scale herds the company envisions.
At the foundation of Colossal’s work is CRISPR. This technology, adapted from a method bacteria evolved to identify attacking viruses and chop up their DNA, is now a mainstay of genetic engineering, and Church has been involved since CRISPR’s earliest days.
There are other ways Colossal hopes to help. Its gene editing technology could artificially add genetic diversity to species with only small surviving populations, Lamm said.
Jurassic Park-style tourism? Nope (?)
Selling or licensing spinoff technology is a somewhat indirect way of running a business. A more direct option is selling tickets to tourists. After all, humans already pay lots of money to see charismatic megafauna like lions, elephants and giraffes on African safaris. Seeing a creature that’s been gone for 10,000 years could add to the excitement.
Another candidate species Colossal wants to re-create is the woolly rhinoceros, a relative to the critically endangered Sumatran rhino.
Although Colossal doesn’t plan to build a tourist destination, it does have a woolly mammoth rewilding site in mind that sounds awfully close to Jurassic Park: Pleistocene Park. This area of about 60 square miles in northern Russia, named after the geologic period that ended with the last ice age, is where researchers Sergey and Nikita Zimov are trying to test their theories about the ecological and climatic effects of rewilding.
One Zimov idea is that woolly mammoths will trample snow and knock down trees. That, in turn, will restore grasslands that reflect more of the sun’s warming rays and eliminate insulating snow and forests so the ground cools more. And that means the ground will stay frozen instead of releasing its current store of carbon dioxide and methane greenhouse gases. About 260 billion to 300 billion metric tons of carbon could be released from thawing permafrost by 2300, scientists calculate, exacerbating the weather extremes and other problems caused by climate change.
There’s an appeal to the idea of de-extinction. Humans have dramatically altered the planet, and the United Nations estimates we threaten 1 million species with extinction as a result.
Colossal hopes its work will raise more attention to the biodiversity collapse. And it also plans to create detailed genetic descriptions of many endangered species “so we have the recipe if that species does go extinct,” Lamm said.
But is that really the best use of our resources to help the planet? No, some researchers believe.
Resurrecting species could have some benefits, but money would be better spent protecting ones that are still around, a group of biologists argued in one paper published in Nature Ecology & Evolution. “Potential sacrifices in conservation of extant species should be a crucial consideration in deciding whether to invest in de-extinction or focus our efforts on extant species,” the researchers wrote.
But this isn’t government money Colossal is talking about, and Lamm argues that his startup’s work complements other conservation efforts. And, he argues, startups can move faster than government-funded work.
Oh certainly, especially with all the force and finance of the NWO GLOBAL GOVERNMENT and its PHONY PHILANTHROPISTS, CORPORATE HEADS, RULING ELITE AND NON-GOVERNMENTAL ENTITIES.
In a world dominated by climate crisis headlines, a startup that makes money with an ecosystem-improvement focus has special appeal. One investor, Zack Lynch of Jazz Venture Partners (JAZZ seeks breakthroughs at the convergence of technology, science, and humanity), is excited by software, hardware and biotech he expects Colossal will create.
At the same time, “these breakthroughs will help address issues such as land degradation, animal pollinator loss and other negative biodiversity trends, (all created by Technology)” Lynch said. Given how big our environmental problems are, you can see why an investor might be interested.
spacer
Just off the coast of Costa Rica lies an island, Isla Nublar, that provides habitation for hundreds of genetically engineered dinosaurs. This small island is, of course, the focal point of the movie, Jurassic World. CRISPR technology is walking the line between science and science fiction, so let’s take a look at whether CRISPR could bring back extinct dinosaurs.
spacer
CRISPR Helps Life Find a Way
We previously covered how CRISPR could be used to create the X-Men and Hawkeye of the Avengers. In this article we turn to the Jurassic Park movie franchise to explore how CRISPR might be able to bring back dinosaurs from extinction.
In the first Jurassic World movie, released in 2015, we revisit the site of the original Jurassic Park where a new theme park has opened, operating under the name “Jurassic World.” The movie opens with geneticist Dr. Henry Wu working to create a hybrid dinosaur to attract visitors. We then follow the park’s staff and visitors as the new hybrid, the extremely intelligent and vicious Indominus rex, [spoiler alert] escapes and sets off a chain reaction that causes the other dinosaurs at the park to roam freely.
This summer we revisit Isla Nublar for Jurassic World: Fallen Kingdom, the second movie in a planned Jurassic World trilogy and the fifth in the Jurassic Park series. Dinosaurs have been roaming the island unhindered for three years, and an impending volcanic eruption threatens their existence. The new movie begins with establishment of a new dinosaur rescue organization which aims to bring the creatures to sanctuary in the USA.
In the original Jurassic Park, DNA was taken from a microscopic drop of dinosaur blood that had been preserved for ~65 million years in the gut of a mosquito trapped in fossilized amber. Some non-specific activities involving Jeff Goldblum, chaos theory, and the yolk of a crocodile egg resulted in a thriving ecosystem made up of once-extinct creatures.
The idea of resurrecting extinct creatures using CRISPR has been discussed at length since the technology was developed. These conversations have largely focused on the woolly mammoth, which last roamed the Earth 3,300 years ago.
In 2015 a team of researchers working at Harvard University took a significant step toward the creation of a creature that would, on an initial approximation, look like a woolly mammoth – a woolly mammoth 2.0 of sorts. Rather than using DNA directly from one of the mammoth carcasses that have been found preserved in ice, the team rebuilt the genome of the woolly mammoth using the genome of an Asian elephant as a base. CRISPR-Cas9 was used to replace 14 loci in the elephant genome, with the mammoth version of those sequences. The edited DNA included genes that affect blood hemoglobin, ear size, subcutaneous fat, and hair.
Extinct Animals Have Already Been Brought Back
Researchers have clearly not yet triggered the live birth of a woolly mammoth, but we have already seen the birth of a living creature previously declared extinct. The Pyrenean ibex, or bucardo goat, was once found in the mountains of northern Spain. The last ever bucardo, affectionately named Celia, died in the year 2000.
In July 2003 a clone of Celia was born. Unfortunately, the clone had a lung defect and couldn’t breathe properly, so it died just 10 minutes after it was birth. The bucardo is now often referred to as “the animal that went extinct twice.”
Using CRISPR to Bring Dinosaurs Back from Extinction
The resurrection of the bucardo goat relied on the ability of scientists to access cells from a living sample. DNA samples from Celia were cryogenically frozen while she was alive to ensure optimal preservation.
We don’t have that option with dinosaurs. So what can be done instead?
Red blood cells and fibers of ancient protein have been found in dinosaur fossils held in the archives of the Natural History in London, UK. However, we now know that readable DNA information can only remain intact for approximately 1.5 million years – not the 75 million years that these fossils have been around for.
Therefore, the “woolly mammoth 2.0” idea may be a starting point for the resurrection of dinosaurs. We know that birds are descendants of dinosaurs, so scientists may be able to use their genome as a base, splicing in genes coding for dinosaur-like traits using CRISPR-Cas9.
Conclusion
Should CRISPR Reverse Extinction?
Resurrecting the woolly mammoth seems much more possible than dinosaurs, but many people are wondering whether we should do it, even if we could. Some experiments have indicated that a mammoth-trampled tundra would keep the ground colder, preventing it from thawing in warmer climates. With climate change becoming a point of increasing global concern, it would appear that bringing back mammoths could offer humans a tangible benefit should we manage to pull off their resurrection.
Bringing back dinosaurs is another story. The Jurassic Park franchise, specifically the second installment of the Jurassic World trilogy, demonstrates that bringing back dinosaurs would likely result in more harm than good. Sometimes science fiction is better off remaining fiction.
Sadly, we have seen over and over again that scientists do not care whether their efforts bring about benefits or destruction. They don’t worry about the risks. They plod on, determined to accomplish whatever enters their sick little minds.
Even if it means opening up portals for demonic beings, causes irreparable damage to our environment or even destroying EARTH altogether in a Gigantic Explosion or a DEEP DARK HOLE.

Repost from August 14, 2022. | Steven Ben-Nun (Israeli News Live) | Let’s examine the resurrection of Nimrod and its implication if the Darkside can pull it off.
My Tidbit: I wonder if Homo Capensis is among us. The late Karen Hudes used to mention them.
Steven Ben-NunPO Box 156 Sunbright TN 37872
—————DISCLAIMER:———————————————Please do your own research and come to your own conclusions. 😉 Thank you! 👍
All footage taken falls under ”fair use” of the Digital Millennium Copyright Act (1998). Therefore, no breach of privacy or copyright has been committed. Copyright Disclaimer, Under Section 107 of the Copyright Act 1976, allowance is made for ‘fair use’ for purposes such as criticism, comment, news reporting, teaching, scholarship, and research. Fair use is a use permitted by copyright statute that might otherwise be infringing. Non-profit, educational or personal use tips the balance in favor of fair use.
spacer

Truth Frequency Radio – 4/30/2017 – Anthony Patch joined us today for part 2 of our look at CERN and the ancient Tower of Babel connection. Is CERN attempting a cosmic jailbreak? Is their agenda to open the abyss as prophesied in the Bible? Will they use the technology to resurrect Osiris/Nimrod as the Antichrist. Are they preparing for war against the Holy Spirit? How is the Vatican involved? What is the Internet of Things and how does it relate to Agenda 2030, Artificial Intelligence, D wave computers, and Enslavement? Are the powers that be terraforming the planet for a new race of immortals? Vaccines? Could they be a precursor to the mark of the beast? All of these questions addressed and more! Plus the August 21st eclipse, Stolen Gold Coins and September 23rd end time markers. Rejoice for the Kingdom of God is at hand! Many thanks to Anthony Patch for joining me! Much Love and God Bless!
website: https://www.anthonypatch.com
Listen LIVE to The Awakening Liberty Show with Sean Caron every Saturday and Sunday at 2 PM Pacific Time!
http://truthfrequencyradio.com/listen…

for education purposes only.
What will happen when “they” control all the food?

LOOK AT WHAT THEY ARE DOING!!


70% of the population has an auto-immune disease, and 99% of people on earth has inflammation at some point during the day. Increased environmental toxins, poor diet, lack of sleep, stress, all cause bacterial imbalance in the gut. Bacterial imbalance in the gut directly affects your immune system.Antibiotics do more to imbalance your gut than almost any other factor. It can take up to six months for your good bacteria (probiotics), making you more vulnerable to disease and infection. The more bacteria is imbalanced, the more bad bacteria can wreak havoc in your body in numerous ways – one of the biggest is the formation of biofilm.Biofilm is responsible for 80% of all disease and infection.The probiotic supplements lining the pharmacy and grocery store isles are not able to make it through the bile system to the colon where they are needed.Additionally, they are incapable of removing biofilm.This is why P2 Probiotic Power Digestive H2O Additive is so important. The spore-based probiotics in this product are hearty warriors that do make it through the bile system to the colon. They can act like an antibiotic when needed and go dormant and then come back when needed.They do these other amazing

spacer
{From A Viewer: Jews are Esau Edomites. 1) Edom is modern Jewry. Jewish encyclopedia 1925 vol. 5 page 41. 2) “Strictly speaking, it is incorrect to call ancient Israelites a Jew or to call a contemporary Jew an Israelite or Hebrew. Jewish almanac 1980 page 3. 3) Edomite Jews began to call themselves Hebrews and Israelites in 1860. Encyclopedia judaic 1971 vol. 10 page 23} * God Said In Last Days All Things Will Be Revealed The Vail Is Coming Off! I Don’t Follow Q I Follow Jesus!
LINKS TO PATENTS OUR GOVERNMENT HAS…LOOK FOR YOURSELF IF YOU DON’T BELIEVE: https://patents.google.com/patent/US6017302A/en
https://www.geoengineeringwatch.org/links-to-geoengineering-patents/ & https://www.geoengineeringwatch.org/
Fallow me on TeleGram Here: https://t.me/SherriLynnMinistries This is the new Direction! * Word of The Day: Revelations 16:15, Revelations 13:18 & 14: 1-5, Matthew 7:12-21, Romans 8 & 9… They are taking away all our Constitutional rights using this COVID 19. * Free Copy of Dossier https://sherrilynn.com/people-resources * going back to the beginning Genesis 1, Wake Up and Pray Saints! Put on “The whole Armor of GOD!” We are working together to save our Country in Jesus Name! Thank you for your support. National Liberty Alliance Know Your Constitution: https://www.nationallibertyalliance.org. Do your research please. United We Stand! If you want Information: sherri@sherrilynn.com or if you want more information: https://sherrilynn.com/my-blogsKnow Your Rights Use This if you are made to get Vax. * Revelations 9:11 And they had a king over them, which is the angel of the bottomless pit, whose name in the Hebrew tongue is Abaddon, but in the Greek tongue hath his name Apollyon. Pray for the children! COP https://en.wikipedia.org/wiki/2022_United_Nations_Climate_Change_Conference
* Fair Use Notice: This video contains some copyrighted material whose use has not been authorized by the copyright owners. I believe that this not-for-profit, educational, and/or criticism or commentary use on the Web constitutes a fair use of the copyrighted material (as provided for in section 107 of the US Copyright Law. If you wish to use this copyrighted material for purposes that go beyond fair use, you must obtain permission from the copyright owner. Fair Use notwithstanding I will immediately comply with any copyright owner who wants their material removed or modified, wants us to link to their web site, or wants me to add their photo. The Vision Host Sherri Lynn channel is “one Women’s opinion”. Anything that is said on the channel is either Bible, Gods Word, opinion, criticism, information orcommentary.
God Bless,


Source: History Channel don’t know who recorded it from there.
People still deny this could be in the COVID 19 vaccines.
Repent and ask Jesus Christ to forgive you. We are in the last days of Bible Prophecy. The time is short.
Just Say No To Drugs. Say No to Mask. Say No to PCR Test.
The COVID 19 Vaccine is the Mark of the Beast.
Have a blessed day!!!

2 Corinthians 2 : 11 “Lest Satan should get an advantage of us: for we are not ignorant of his devices.”
GET SAVED NOW !!1 Corinthians 15:1-4“ 1 Moreover, brethren, I declare unto you the gospel which I preached unto you, which also ye have received, and wherein ye stand;2 By which also ye are saved, if ye keep in memory what I preached unto you, unless ye have believed in vain.3 For I delivered unto you first of all that which I also received, how that Christ died for our sins according to the scriptures;4 And that he was buried, and that he rose again the third day according to the scriptures ”
Romans 10:9-10“ 9 That if thou shalt confess with thy mouth the Lord Jesus, and shalt believe in thine heart that God hath raised him from the dead, thou shalt be saved.10 For with the heart man believeth unto righteousness; and with the mouth confession is made unto salvation. “
Romans 10 : 13 “ For whosoever shall call upon the name of the Lord shall be saved. “
2 Timothy 2 :19 “ Nevertheless the foundation of God standeth sure, having this seal, The Lord knoweth them that are his. And, Let every one that nameth the name of Christ depart from iniquity. “
Copyright Disclaimer under section 107 of the Copyright Act 1976, allowance is made for “fair use” for purposes such as criticism, comment, news reporting, teaching, scholarship, education and research. Fair use is a use permitted by copyright statute that might otherwise be infringing. Non-profit, educational or personal use tips the balance in favor of fair use.

“Rules For Thee, But Not For Me!”
King Charles just gave his royal assent to new UK laws that allow gene-edited foods to go unlabeled.
Despite having a personal, strictly organic food supply for himself.

They don’t need to microchip you, to connect your body to the ‘Cloud’.
spacer


Hacking the software of life…They have been at this for a long time. Now they are using it on the the human race! This is an extinction level event that we must stop at all cost!THE GLOBAL ELITE ARE PEDOPHILES

December 19th, 2022.
Things are getting very dire. The stock market is taking a huge HIT and the evils are spreading the word “recession.” which means people won’t spend money, more jobs lost, more businesses will shut down and more people will be starving.
Actions speak louder than words!!!
For Serious People Worldwide who want to join or build an offline community based on FREEDOM to live on. Join the OFS Community platform.
I give credit to each channel I got the video from if I go it from one of the 4 video platforms, as sometimes I get the videos from Telegram or FB so there is no link.
For both Truthers and Normies… the ONLY way we are going to fight this thing is IF you finally recognize that you have power within and we ALL work together.
Please PLEASE start releasing your childhood trauma. We all have some.
This is why it’s so easy for the evils to control us, because they have harmed us psychologically for hundreds of years through all sorts of trauma – war, poverty, death, sickness through their poisons, physical and sexual abuse, all sorts of EMFs, RFs, MFs, etc., AND brainwashing and mind control.
Then our parents pass that trauma down onto us and unless you clear most of it, you will pass it to your children and so on.
Then we need to learn how to tap into our inner power and reach our higher self.
Looking outside yourself is what they want you to do so you think you have NO POWER.
Also, so long as our vibration is constantly low, they can bring us further down, so we all need to learn how to raise it as much as possible.
I know it’s not something we can do all of the time unless we are in a loving joyful state, but we need to protect ourselves from these evils.
My main video channels
I’d also like to hear your thoughts on the video with a comment.
We MUST work together to not only save us from this war, but also change our world completely. We can’t do it without you.
And if you can help support our work which I’ve been doing full time for free since the war started, that would be much appreciated. You can even just click on links and buy things you need so I get a commission. It costs you nothing other than what you needed anyway.

The Long Pause Is At Its End.

The Jab uses technology that permanently alters humanity. This is the transhuman agenda and biblical prophecy of Daniel about mixing iron with clay and mingling seed.

DARPA HYDRO-GEL QUANTUM-DOT LUCIFERASE- CELESTE SOLUM – #MARKOFTHEBEAST
——————————————————————–
GLOBAL ZIONIST NAZI COMMUNIST TAKE OVER !!!!#BEASTSYSTEM#PERSECUTION#TRIBULATION#GEORGIAGUIDESTONES#TARGETEDINDIVIDUALS#GENOCIDE#MARKOFTHEBEASTVACCINE#TARGETEDINDIVIDUALS#5G #DIRECTEDENERGYWEAPONS#CRIMESAGAINSTHUMANITY
#TARGETEDINDIVIDUALS BLUE RAVEN


Crisper, mRNA, “hacking the software of life”…they have been at this for a long time. Now they are using it on the the human race! This is an extinction level event that we must stop at all cost!

Pfizer and CRISPR – The road to hell is paved with good intentions. In the first few weeks of 2022, the $285 billion New York pharma company Pfizer announced deals with two gene-editing startups. Beam Therapeutics and Mammoth Biosciences.

From the report of these GMO babies which is way out there, I looked into the Trans-humanism agenda using the Crisper9 Technology. China has been doing this for many years and also the USA, France & Russia.This reminds me of the Genesis ch. 6:4 account where the sons of God came in unto the daughters of man, and they bare children to them, the same became mighty men which were of old, men of renoun.
But as the days of No’e were, so shall also the coming of the Son of man be.Matthew 24:37
spacer

spacer

First published at 20:18 UTC on November 10th, 2023.Not Fake News
spacer

If you have not viewed the following posts, be sure to check them out.
Great RESET – Internet of Bodies
AIOT will TOTALLY CHANGE EVERYTHING
DIGITAL LOCK DOWN of EVERYTHING
DIGITAL ID and the Sentient Universe – Part 1
DIGITAL ID and the Sentient Universe – Part 2
Not my post but A VERY GOOD READ, WORTH YOUR TIME:
The Dark Side of CRISPR
Feb 16, 2021 — Its potential ability to “fix” people at the genetic level is a threat to those who are judged by society to be biologically inferior. Source